Chronic kidney disease (CKD) is a worldwide public health problem. The overall prevalence of CKD in the US adult population was 14.8%, using an estimated glomerular filtration rate of <60 ml/min/1.73 m2 as a definition for CKD.1,2 The prevalence of end-stage renal disease (ESRD) continues to increase. According to the US Renal Data System, the incidence rate is 357 per million per year.3 Of these ESRD patients, 63% were receiving haemodialysis, 7% peritoneal dialysis and 29.6% had a functioning kidney transplant.3
Patients with ESRD need long-term vascular access for haemodialysis. The most commonly used vascular access is the arteriovenous shunt (AVS). The AVS is a connection between the arterial and venous systems created either using an anastomosis between a limb artery and superficial native vein (arteriovenous fistula; AVF) or insertion of graft (arteriovenous graft) as dialysis access, creating a left-to-right shunt.4
The presence of an AVS has a significant haemodynamic impact on the cardiovascular system – both short- and long-term. It is a common cause of high-output cardiac failure. The mechanism underlying this haemodynamic effect is based on shunting blood from a high-pressure artery via the AVF to a low-pressure vein, thus bypassing capillary beds and decreasing systemic vascular resistance (SVR). These haemodynamic changes stimulate a compensatory increase in heart rate, stroke volume and total plasma volume.5 The elevation in cardiac output (CO) associated with AVS depends upon the size of the shunt and the magnitude of the resultant reduction in SVR. Because blood flowing through the shunt bypasses the capillary circulation, the total CO increases by the quantity of blood flowing through the shunt to maintain capillary perfusion.6
In high-output heart failure (HF), low SVR results in borderline preserved or depressed systemic arterial blood pressure and elevated cardiac filling pressures. Ineffective blood volume and pressure lead to activation of the sympathetic nervous system and the renin–angiotensin–aldosterone axis along with increased serum vasopressin (antidiuretic hormone) concentrations. This neurohormonal activation results in increased renovascular resistance and reduced renal blood flow and glomerular filtration rate, with retention of salt and water. Chronic volume overload may gradually cause ventricular enlargement, remodelling and HF.6
Definition of Heart Failure with Preserved Ejection Fraction
HF with preserved ejection fraction (HFpEF) is a clinical syndrome in patients with current or prior symptoms of HF with a left ventricular ejection fraction (LVEF) ≥50% and evidence of cardiac dysfunction as a cause of symptoms (e.g. abnormal LV filling and elevated filling pressures).7,8 Patients with HFpEF represent half of all HF patients worldwide. The remaining half have an LVEF <50%, which includes HF with reduced ejection fraction (HFrEF; LVEF ≤40%) and HF with mid-range ejection fraction (LVEF 41–49%).9,10
History of the Arteriovenous Fistula and Relation to Heart Failure
The AVF was first described and used as a reliable form of haemodialysis vascular access by Brescia et al. in 1966.11 Improvements in dialysis technology and the expansion of dialysis eligibility (for example the inclusion of patients with diabetes) resulted in rapid growth of the ESRD population. Many of these patients benefited from the development of prosthetic grafts when autogenous AVFs were not feasible.
In the mid-1980s, the use of permanent catheters (central venous catheters; CVCs) in the internal jugular vein dramatically increased. The cumulative effect was a decrease in AVF use and an increase in graft and CVC use in the 1990s.12 This was associated with increased patient care costs; for example up to 73% of patients were hospitalised to initiate dialysis and almost invariably had a temporary CVC inserted.12,13 This led the Centers for Medicare and Medicaid Services and National Kidney Foundation in the US implementing in 2003 the Fistula First Initiative to increase AVF placement and use to 65% along with lowering costs.13 AVF is still the best choice for dialysis access in terms of patient outcomes/survival and reducing health care cost, but the approach can be associated with complications.
Incidence of Heart Failure Post-arteriovenous Shunt
Studies have shown that an estimated 17–26% of patients with a functioning AVS develop symptoms of HF.14,15 Factors associated with AVS precipitating HF include development of right ventricular dilatation, left atrial dilation, development of AF, male sex, prior vascular access surgery and high haemodialysis arteriovenous access flow rate.16 The risk of worsening HF is directly proportional to the flow of the haemodialysis arteriovenous access and is greater with pre-existing poor cardiac function.17 There is no threshold access flow rate that defines risk. Even what is considered to be a normal flow may worsen or precipitate HF in patients with pre-existing HF or heart disease.16
The risk of precipitating HF appears to be higher among patients who have an upper-arm AVF compared with forearm AVF.14,15 The higher risk associated with upper-arm AVFs appears to be related to higher blood flow. In an observational study of 562 pre-dialysis patients, the incidence of HF was much higher in patients who had a brachiocephalic AVF compared with those with a radial-cephalic AVF (40% versus 8%).14 Similar rates of HF have been observed among patients with AVFs compared with those with arteriovenous grafts.16 There are no data to assess whether the technique used to create the AVF (direct versus through translocation or transposition) has any relation with the development of HF.
Haemodynamic Changes After Arteriovenous Shunt Creation
The creation of AVS results in acute, sub-acute and chronic cardiovascular changes.
Acute Changes
Acute effects of AVS creation include an immediate decrease in SVR and consequent increases in forward stroke volume, heart rate and CO. The decrease in total SVR is the result of both changes in the vessels associated with the arteriovenous access (called access resistance) and changes in other systemic vessels. In response to increases in blood flow and shear stress, the vascular endothelium releases nitric oxide and other endothelium-dependent relaxing factors that dilate the artery, reducing shear stress towards normal.5,18
The decrease in SVR causes an acute fall in both central and peripheral blood pressure. In response, there is an increase in sympathetic nervous system activity (which increases contractility and heart rate). It is this combination of decreased cardiac afterload and increased sympathetic activation that causes acute increases in CO.5 The CO increases immediately upon creation of the AVS and continues to increase over time.19,20 This increase in CO leads to an increase in venous return to the right side of the heart, leading to right ventricular dilatation in some patients.16 Conversely, acute compression of AVS increases the SVR and blood pressure and decreases CO. The increase in blood pressure leads to baroreceptor-reflex-mediated reduction in heart rate (Nicoladoni-Branham’s sign; Figure 1).
Subacute and Chronic Changes
Subacute changes occur within days after creation of the AVS. Within 2 weeks of AVS creation, blood volume increases, leading to greater venous return and increased right atrial, pulmonary artery and LV end-diastolic pressures. Both plasma atrial natriuretic peptide and brain natriuretic peptide concentrations increase after AVS creation, peaking 10 days postoperatively.6,21 CO continues to increase over days and weeks after creating of the AVS.19,20
Many studies have sought to understand the effect of AVF creation or closure on heart structure and function. Most use non-invasive methods – mainly echocardiographic parameters – while others use Doppler ultrasound to assess AVF flow and its effect on LV parameters. One study used cardiac MRI as an accurate non-invasive tool for the assessment of cardiac functions and dimensions.4 In 2018, Saleh et al. published a study investigating patients with AVFs and HF.22 The study showed that higher AVF flow is associated with an increased risk of high-outflow HF (HOHF). Furthermore, the study demonstrated a strong relationship between the amount of AVF flow (Qa) in relation to CO and the development of HOHF. A Qa/CO ratio ≥20% was an independent predictor of HOHF.22 Table 1 summarises the key studies evaluating the effects of AVF on the heart.
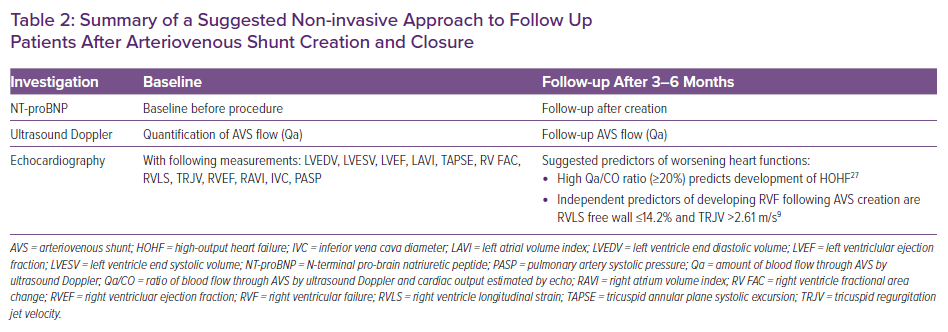
Cardiac Follow-up After Creation of an Arteriovenous Shunt
Evaluation of all patients following an AVS includes an evaluation for HF. All patients who undergo access placement have markedly reduced kidney function and are at risk for HF. Patients who are at particular risk to develop HF related to the arteriovenous access include those with a large distended AVS, especially in the upper-arm position.20,21
Monitoring Strategies
Patients should be followed for signs and symptoms of HF as a routine part of every visit to determine whether HF is present. An echocardiogram should be obtained when any new symptoms or signs suggestive of cardiac dysfunction develop, and follow-up echocardiography 3–6 months after creation of the AVS is also recommended. Echocardiographic findings suggesting the development of HF include dilation of the inferior vena cava, new right ventricular dilation or dysfunction and increasing estimated pulmonary artery pressures.16,23
The AVS should be examined at every visit. The presence of a large, distended fistula with very strong thrill is suspicious for high blood flow and should prompt a quantitative evaluation, particularly in the presence of HF signs and symptoms. Patients with a calculated blood flow through the AVS by ultrasound Doppler (Qa) >2 l/min are at increased risk for the development of HF. Blood flow >2 l/min may predict the occurrence of high-output HF.23 However, Qa ≤2 l/min does not exclude AVS-induced HF. Table 2 summarises suggested non-invasive approaches to the follow up of patients after AVS creation or closure.
Approach to Diagnosis of HFpEF After Arteriovenous Shunt Creation
In patients with AVS who are diagnosed with new-onset or worsening HF, it is recommended to obtain a comprehensive echocardiogram (with assessment of ejection fraction and CO) and to non-invasively measure AVS blood flow by ultrasound Doppler. The presence of one or more of the following echocardiographic findings is suggestive of arteriovenous-access-related HF: dilation of the inferior vena cava, right ventricular enlargement or dysfunction, elevation in estimated pulmonary artery pressures or LV enlargement.6
For patients with an AVS who have new or worsening HF with supportive findings on echocardiography, invasive evaluation of cardiac haemodynamics by right heart catheterisation at rest and with transient fistula occlusion can be helpful. This approach allows the definitive assessment of volume status, direct determination of CO and pulmonary artery pressures and examination of the haemodynamic response to transient fistula occlusion.
Transient fistula occlusion (30 seconds) can provide valuable data when considering management strategies. Transient fistula occlusion should produce a reduction in CO that is often coupled with reduction in central venous pressure. Pulmonary artery and pulmonary capillary wedge pressures may not decrease during transient fistula occlusion because of the acute increase in cardiac afterload.24
Some studies have suggested assessing the cardio-pulmonary recirculation value, which is the ratio of arteriovenous access flow (Qa) to the CO in patients with arteriovenous access flow >2 l/min. A Qa:CO ratio >0.3 indicates a significant risk of developing high-output cardiac failure. However, a Qa:CO ratio ≤0.3 or a Qa ≤2 l/min does not exclude access-related HF.24
Examination and Transient Occlusion of the Arteriovenous Shunt
The presence of a large, distended AVS with very strong pulse augmentation suggests high volume flow and should prompt an evaluation to determine effect of the access on systemic haemodynamics. When the AVS is transiently occluded, the degree of the arterial pulse increase (augmentation) distal to the AVS anastomosis is proportional to the AVS flow.
Transient maximal occlusion (sphygmomanometer inflated to 50 mmHg above systolic pressure for 30 seconds) of a haemodynamically significant arteriovenous access usually decreases heart rate, raises arterial pressure, and lowers venous pressure; this has been termed the Nicoladoni-Branham sign. The Nicoladoni-Branham sign has been shown to be related to arterial baroreceptor activation and increased arterial baroreflex sensitivity.25 In addition to a decrease in heart rate, there is also an increase in arterial blood pressure and increase in SVR, lead to a decrease in CO. Presence of a Nicoladoni-Branham sign was found to be predictive of reduction in LV hypertrophy after AVF ligation.26
Right Heart Catheterisation in Patients with Arteriovenous Shunt
Among patients with AVS, the contribution of the AVS to pulmonary artery hypertension can be initially assessed by manually compressing the AVS under heparinisation and a tourniquet set to at least 30 mmHg above systolic blood pressure for 1 minute, while measuring pulmonary haemodynamics on right heart catheterisation. If a significant component of the patient’s pulmonary artery hypertension is related to the AVS, the mean pulmonary artery pressure, right atrial pressure, and possibly the pulmonary capillary wedge pressure and LV end diastolic pressure will significantly decrease (and even normalise) by at least 20% when the arteriovenous access is compressed. However, the definition of what constitutes a significant decrease is not established and is highly subjective. There may be some concern that such compression will lead to thrombosis of the access, particularly if the access is an arteriovenous graft. However, in practice, it is much harder to thrombose an arteriovenous access with manual compression than one would expect.27
Management of HFpEF in Patients with Arteriovenous Shunt
In patients with AVS-related HF, management begins with control of volume status with dialysis and diuretics, correction of anaemia, treatment of hypertension and pharmacological management of HF. If HF remains uncontrolled despite medical therapy, the following approach is suggested:
- Close any unused AVS. If the patient has more than one arteriovenous access, one should be closed immediately if it is thought to be contributing, with preservation of the shunt with the best blood flow. The patient’s clinical status should then be reassessed.
- If refractory HF persists with absence of an unused AVS, reduce blood flow of the AVS as close as possible to minimum volume flow necessary for adequate dialysis (600 ml/min). Several different surgical techniques have been used to reduce AVF flow. The goal of surgery is to reduce fistula blood flow while maintaining sufficient flow for adequate dialysis. These techniques have included access banding and plication or distalisation of the anastomosis to a smaller artery.28–30 In one study of 12 patients with a high-flow AVF and clinical signs of high-output HF, a precision banding procedure was effectively used for access flow reduction.29 Adequacy of access flow restriction was evaluated intraoperative using ultrasound flow measurements, adjusting the banding diameter in 0.5 mm increments to achieve the targeted AVF flow. Mean access flow was reduced to a mean of 598 ml/min (481 to 876) after banding. The clinical signs of HF disappeared, and AVFs remained patent in all patients. Two patients had renal transplant failure and later successfully used the AVS. Follow-up post banding was 1–18 months (mean = 12).29
- If refractory HF persists, occlude the AVS. If the approach defined above is ineffective, the AVF should be occluded and replaced with a tunnelled catheter or small graft since the resistance is higher in grafts than greatly dilated fistulas. Peritoneal dialysis may also be an option among some patients.29
Conclusion
The presence of AVS in ESRD patients carries a significant impact on cardiac functions, especially in patients with reduced cardiac reserve (HFrEF or HFpEF). It can precipitate HF decompensation in the short term or long term. The available data on the effect of AVS creation on worsening of HFpEF are limited, with most focused on HFrEF and conducted using non-invasive imaging techniques such as echocardiography or cardiac MRI). Using right heart catheterisation – the gold standard for assessment of haemodynamics and intracardiac pressures – to evaluate the haemodynamic effects of AVS creation or closure may provide more valuable information.