Heart failure with preserved ejection fraction (HFpEF) is a complex clinical syndrome affecting approximately 3 million people in the US and up to 32 million people worldwide. Patients with HFpEF are hospitalised approximately 1.4 times per year and have a mortality rate of approximately 15% per year.1
HFpEF is mainly characterised by the presence of diastolic dysfunction (DD) and elevated left ventricular (LV) filling pressure, in the setting of a LV ejection fraction (LVEF) ≥50%.2 Despite epidemiological evidence of a steadily increasing prevalence, both in absolute terms and relative to the entire HF population, a prompt diagnosis of HFpEF is still challenging. Two scores have been created to better recognise this syndrome: the 2018 H2FPEF score and the 2020 HFA-PEFF algorithm.3,4 Although they represent a remarkable step forward for a correct diagnosis, this approach still leaves many cases in the ‘uncertainty area’.
From a haemodynamic perspective, a hallmark of this syndrome is a pulmonary capillary wedge pressure of ≥15 mmHg (at rest) or ≥25 mmHg (after exercise), or LV end-diastolic pressure ≥16 mmHg (at rest). However, direct haemodynamic measurement during exercise is not widely available; it is an invasive procedure and should be kept for very selected cases. Right heart catheterisation at rest and during exercise is indeed the diagnostic gold standard for an accurate HFpEF diagnosis.5
HFpEF is mainly characterised by different phenotypes driven by different cardiac and non-cardiac comorbidities. This is probably the reason why several HFpEF clinical trials did not reach strong outcomes to recommend a single therapy for this syndrome, as happened in heart failure with reduced ejection fraction (HFrEF), where four pillars have been elected as optimal medical therapy from international guidelines.6,7 Recently, the paradigm has changed and the unmet clinical need for HFpEF treatment found a proper response as a result of a new class of drug, the sodium–glucose cotransporter 2 inhibitors (SGLT2i), which beneficially act through the whole spectrum of LVEF.8
In this review, we focus on the HFpEF phenotypes according to the latest evidence, the role of the new drugs and the potential role of new devices to treat this complex syndrome.
The Origin of HFpEF Phenotypes
The term ‘preserved ejection fraction’ was first coined in the CHARM program to split the enrolled population into two subgroups on the basis of the most validated and readily available tool to define LV squeezing function, which was LVEF measured by echocardiography.9 This ‘heart-centred’ approach proved to be very effective in defining the subgroup of patients who could benefit from therapies targeting neurohormonal activation.
The mainstays of the reduced ‘LVEF paradigm’ consisted of: a single organ involved, or at least responsible for the clinically relevant outcomes; an easily recognisable dysfunction, with no need to deeply investigate the underlying aetiology, at least for what concerns the basic pharmacological treatment; and effective therapies approved by international guidelines to treat the disease.
Similarly, scientific communities tried to apply the same ‘paradigm’ to patients with a normal LVEF. Nonetheless, this model did not fit in this setting as: multiple organs and systems are involved, with the heart playing an important, but not exclusive, role due to cardiac and non-cardiac comorbidities; a variety of morphological and functional abnormalities of uncertain significance, with rest echocardiography still playing a key role as a gatekeeper, but with the need to implement it with other techniques; and frequent inconsistency between phase II clinical trials showing improvement in surrogate outcomes and neutral phase III clinical trials with neutral results on clinically relevant outcomes.
All of this evidence led us to consider the importance of phenotype in HFpEF, to address a tailored therapy rather than ‘one size fits all’.
Phenotyping for Tailored Therapy
Complexity and multimorbidity are the hallmarks of HFpEF. This has been graphically represented as overlapping circles, where every circle is a pathophysiological abnormality.10 According to this model, patients with HFpEF could fall into one or another part of the circle and they could develop the syndrome on the basis of a single mechanism or as the result of multiple concurrent injuries. Addressing the single components of the picture seems to be the way for tailored treatment.
In contrast, the clinical phenotypic approach is a different and perhaps more ambitious way to classify patients.11,12 The underlying assumption is that HFpEF is not a single disease, but a group of different disease processes that share HF symptoms and signs as clinical manifestation. The studies supporting this view divide HFpEF cohorts into mutually exclusive subgroups, the so-called phenotypes, on the basis of the clinical and epidemiological characteristics of patients.13–20 The cluster of comorbidities characterising a specific phenotype is the result of a specific pathological pathway that could be hopefully targeted by specific treatments.
HFpEF Phenotypes: Pieces of a Complex Clinical Puzzle
The physiopathological complexity and clinical heterogeneity of HFpEF have prompted cardiologists to use artificial intelligence and machine learning techniques to analyse the complex relationships within this diverse population, and identify specific subgroups with the goal of greater internal homogeneity to treat them in the same way. The first research group to attempt this ambitious goal was that of Shah et al., who identified patient subgroups based on their comorbidities, including those related to obesity, metabolic syndrome and pulmonary hypertension.11,21
More recently, Uijil et al. proposed a model consisting of five clusters obtained from HFpEF patients recorded in the Swedish Heart Failure Registry, and validated it externally with the Chronic Heart Failure European Society of Cardiology guideline-based Cardiology Practice Quality project registry.18
The first cluster is that of young patients, with an average age <60 years and a reduced burden of comorbidities, including arterial hypertension and obesity. This group of patients also includes those with HF with improved ejection fraction (EF). Weight loss and blood pressure control using renin–angiotensin–aldosterone system (RAAS) inhibitors (RAASi) are central to these patients.
The next four clusters include a population that is, on average, older (aged between 60 and 90 years), with a higher burden of comorbidities, including arterial hypertension. The second cluster is the most represented and consists of patients with an average age of 77 years who have AF, in the absence of diabetes. These patients, even more than others, benefit from a lenient heart rate control to avoid further reducing their already compromised chronotropic reserve. The third cluster is the second most represented and consists of the oldest patients, with an average age of 88 years, the highest burden of comorbidities and the highest concentration of natriuretic peptides. These patients present with frank congestion, which must be attenuated using diuretics, including anti-aldosterone agents, which have shown remarkable clinical benefit in the TOPCAT trial.22 These patients derive the highest prognostic benefit from the intensive treatment of all comorbidities.
The fourth cluster consists of patients with an average age of 71 years, AF and diabetes. In this group of patients, in addition to glycaemic control, the introduction of an SGLT2 inhibitor appears to be fundamental, as the EMPEROR-Preserved and DELIVER trials have shown a reduction in cardiovascular (CV) mortality and hospitalisation for HF.
Finally, the fifth cluster is the fusion of the second and fourth clusters because it includes elderly patients with an average age of 82 years, diabetes and AF. The DECLARE-TIMI 58 trial found that diabetes patients without AF may benefit from the use of SGLT2i because they have a preventive effect on this arrhythmia.23–25
Beyond the different clusters identified by Uijil et al. and by the countless attempts of many other authors, obesity is a common comorbidity in many patients with HFpEF. Excess visceral adipose tissue promotes the hyperactivation of neurohormonal systems that, together with the reduced plasma activity of neprilysin typical of these patients, make the pro-inflammatory and fibrotic effect of aldosterone unopposed, favouring diastolic dysfunction that is responsible for the progressive increase in filling pressures.21 Considering the typical pathophysiological changes of the obese phenotype, RAASi, particularly aldosterone antagonists and neprilysin inhibitors (ARNI), play a central role, in addition to the use of SGLT2i. Furthermore, the STEP-HFpEF trial has recently demonstrated in obese HFpEF patients that semaglutide versus placebo significantly reduced body weight, improving symptoms, physical limitations and exercise function, with a positive impact on quality of life (QoL), inflammatory status and survival.26
Current Progress in ‘Phenomapping’: Insights From the Literature
Recently, a review conducted by Peters et al. identified the three most recurrent HFpEF phenotypes in studies that focused on specific characteristics through statistical analysis of large datasets, known as ‘phenomapping’.27 These three phenotypes were also observed in studies where researchers did not employ this static strategy, demonstrating some robustness in the results (Figure 1).28 The first two analysed phenotypes closely reflect everyday clinical practice, as they represent two patient groups commonly encountered in outpatient settings. The most prevalent phenotype is ‘older, vascular ageing’, which aligns with Shah et al.’s findings, describing a group of elderly patients with arterial stiffness leading to hypertension and chronic kidney disease.11 These patients exhibit concentric LV hypertrophy on echocardiograms, a higher incidence of chronic kidney disease and elevated levels of inflammatory molecules in the blood, contributing to the pathophysiology of this phenotype and partially justifying its partial overlap with the ‘metabolic, obese’ group.
Additionally, inflammation is responsible for remodelling the left atrial muscle and conduction tissue, promoting the progression of these patients towards ‘left atrial myopathy’, associated with increased pulmonary pressures and right ventricular dysfunction, defining a subtype of this first phenotype. Given that arterial stiffness and kidney disease play a role in the already poor prognosis of these patients, it is evident that blood pressure control with drugs targeting the RAAS plays a central role. Moreover, these elderly hypertensive patients are at a higher risk of AF, which is deleterious in HFpEF patients.
The second most frequent phenotype is the ‘metabolic, obese’, represented by patients with high body weight and type 2 diabetes, predisposing them to widespread atherosclerotic vascular disease, particularly affecting the renal vessels, which accounts for the high prevalence of chronic kidney disease in this group. Obesity leads to increased renin–angiotensin activity and aldosterone levels, explaining why patients with characteristics related to this phenotype showed better responsiveness to spironolactone in the TOPCAT trial.29
While the patients in the first phenotype are older, those belonging to the third phenotype are ‘relatively younger’, with lower levels of stress biomarkers, such as B-type natriuretic peptide (BNP)/N-terminal fraction of pro-BNP (NT-proBNP) and soluble ST2. Identifying these patients solely through peptide assays and resting echocardiography is challenging; thus, prompt additional investigations are necessary, including measurements of telediastolic filling pressures and non-invasive or invasive assessment of pulmonary artery pressures during exercise. Uncertainty remains regarding this third phenotype, which seems to benefit from spironolactone treatment (based on post hoc analysis from the TOPCAT trial in both the full and the Americas cohort) and exhibits some degree of overlap with the ‘metabolic, obese’ phenotype, partially justifying the responsiveness to mineralocorticoid receptor antagonists and lower atrial natriuretic peptide concentrations.29
While older HFpEF patients often experience classic symptoms, such as dyspnoea and fatigue, younger patients with low BNP levels may present with other symptoms, such as exercise intolerance, unexplained dyspnoea on exertion or other non-specific complaints. Medications, lifestyle modifications and interventions that have been shown to benefit older HFpEF patients may need to be tailored for this group. Moreover, traditional hard endpoints, such as mortality, may not be suitable for assessing the efficacy of treatments in younger HFpEF patients. Alternative outcome measures, including QoL, exercise capacity and early markers of HFpEF progression should be considered in clinical trials.
Based upon these considerations, it appears clear that the scarcity of dedicated research focused on ‘young-low BNP populations’ in HFpEF highlights a significant research gap. Indeed, more studies are needed to better understand the unique aspects of HFpEF in this population, and to identify effective diagnostic and treatment approaches to solve this unmet clinical need.
In summary, among the three clinical phenotypes, there is a gradient of age, inflammation, diagnostic tools and poor prognosis, which is highest in the ‘older, vascular ageing’ phenotype and gradually decreases from the ‘metabolic, obese’ to the ‘younger, low BNP’ phenotype.
A New Drug for HFpEF: SGLT2i
In recent years, cardiologists have added SGLT2i to their pharmacological arsenal.6,7 Initially, these molecules were shown to reduce the incidence of CV events, including HF, in patients with type 2 diabetes who were at high or very high CV risk.25,30–32 Subsequently, two randomised controlled clinical trials, DAPA-HF and EMPEROR-Reduced, were conducted with patients with HFrEF.33,34 In these trials, dapagliflozin and empagliflozin, respectively, significantly reduced the incidence of fatal CV events and hospitalisation for HF, leading to their inclusion as the ‘fourth pillar’ of HFrEF pharmacological therapy. The efficacy of these molecules was also studied in HFpEF and heart failure with moderately reduced EF (HFmrEF) in the EMPEROR-Preserved and DELIVER clinical trials.23,24
Both of these trials had positive results, with a significant reduction in the primary outcome (a composite of CV death and hospitalisation for HF). The magnitude of the effect was similar in EMPEROR-Preserved (HR 0.79; 95% CI [0.69–0.90]; p<0.001) and in DELIVER (HR 0.82; 95% CI [0.73–0.92]; p<0.001). The positive result was mainly driven by a lower risk of hospitalisation for HF and worsening HF events. The effect on CV deaths was almost significant in both trials (HR 0.88; 95% CI [0.74–1.05] in DELIVER; HR 0.91; 95% CI [0.76–1.09] in EMPEROR-Preserved). Given the large number of patients needed to reach a significant result, a prespecified meta-analysis of DELIVER and EMPEROR-Preserved was planned, and it proved a significant reduction in CV mortality (HR 0.88; 95% CI [0.77–1.00]).35 SGLT2i also improved health status and QoL, as measured by the Kansas City Cardiomyopathy Questionnaire.36,37
In summary, empagliflozin and dapagliflozin significantly reduced the incidence of CV events and worsening HF in HFpEF, demonstrating that SGLT2i improved the prognosis of HF patients across the entire LVEF spectrum.
The introduction of SGLT2i has opened up a new avenue for HF treatment beyond neurohormonal activation control. The mechanism of action of this drug class is complex and not yet fully understood. However, it is known that inhibiting SGLT2 at the proximal convoluted tubule level causes glucose and sodium to be excreted in the urine, which, due to osmotic effects, promotes diuresis.38 This diuresis, however, is different from that caused by loop diuretics, as it appears to have a selective effect on interstitial volume, with minimal impact on vascular volume. This leads to a reduction in preload and, albeit modestly, blood pressure without triggering compensatory activation of the RAAS.39
Additionally, the increase in sodium concentration in the ultrafiltrate promotes the restoration of a normal tubule-glomerular feedback, which, through the macula densa, induces vasoconstriction of the afferent arteriole. This leads to a reduction in intraglomerular pressure, which, due to haemodynamic effects, reduces the extent and rate of the glomerular filtration rate and proteinuria reduction.40–42 The beneficial effect on the kidneys is purely haemodynamic and visible from the beginning of drug administration. Simultaneously, a transient mild reduction in the glomerular filtration rate and, equally slightly, an increase in creatinine levels can be observed. SGLT2i reduce insulin resistance and contribute to weight loss, both for their diuretic effect and, over time, for the loss of calories in the urine, which induces a fasting-like state promoting lipolysis and ketogenesis.43
Ketone bodies are an alternative source of energy to glucose and fatty acids, improving metabolic efficiency. This is even more evident in patients with HFpEF, whose cardiac cells become less efficient in terms of oxidative metabolism and become increasingly dependent on glycolysis.44 Therefore, ketone bodies provide the cardiomyocyte with an ‘extra’ energy supply without interfering with glucose oxidation, causing improved cardiac performance, regardless of the presence of diabetes.45
SGLT2i: Practical Implications
The introduction of SGLT2i has transformed the paradigm of HFpEF treatment.46,47 Rather than solely targeting neurohormonal activation, this class of drugs addresses comorbidities, which is a peculiar feature of HFpEF, and offers a valuable opportunity for cross-sectional intervention on the cardio–renal–metabolic continuum, which is profoundly altered in these patients.
According to new evidence, SGLT2i should be introduced during hospitalisation for acute HF.48 Clinical studies have shown that their use is safe, well-tolerated and effective in achieving faster decongestion. Furthermore, their use in combination with ARNI and mineralocorticoid receptor antagonists (MRA) has an additive effect, facilitating faster titration of the latter due to improved potassium control.49,50
Neurohormonal Antagonism
Patterns of neurohormonal activation are different in HF cohorts according to LVEF.51,52 Sympathetic and RAAS activation, resulting in elevated levels of plasma renin activity, aldosterone, catecholamines and NT-proBNP, is a key feature of HFrEF. Just a small proportion of HFpEF patients display the same cascade of activation, and the prognostic impact of elevated plasma level of neurohormones (well established in HFrEF patients) is uncertain in the HFpEF subgroup.53
The heterogeneity of the HFpEF population is at least in part responsible for the puzzling results of the several neutral trials that tried to replicate the success achieved in the HFrEF trials.54,55
Angiotensin-converting Enzyme Inhibitors and Angiotensin Receptor Blockers
Three large-scale randomised clinical trials tested the safety and efficacy of angiotensin-converting enzyme inhibitors in HFpEF.56–58 Two of them, the CHARM-Preserved and the I-PRESERVE used an angiotensin receptor blocker (ARB), the PEP-CHF trial used an angiotensin-converting enzyme inhibitor.56–58 CHARM-Preserved inclusion criteria were: LVEF >40%, New York Heart Association (NYHA) class II–IV and previous hospital admission for HF. The primary outcome of composite CV death and HF admission was not met (HR 0.89; [95% CI 0.77–1.03]; p=0.118). The echocardiographic CHARM substudy proved that only half of patients enrolled had a moderate or severe DD, given that a worse DD was a strong prognostic predictor.59 Based on these observations, the clinical trials that followed tried to target a more selected cohort, adding in the inclusion criteria both echocardiographic evidence of DD and congestion. The results were fairly similar, confirming no effects on mortality and some effect on HF hospitalisation. A large metanalysis that included the aforementioned clinical trials and other smaller size studies concluded that both ARB and angiotensin-converting enzyme inhibitors have no effect on all-cause mortality (HR 1.01; 95% CI [0.92–1.11]), CV death (HR 1.02; 95% [0.90–1.14]) and HF hospitalisation (HR 0.92; 95% CI [0.83–1.02]).60
A subanalysis of the CHARM study examined LVEF as a continuous variable and found a significant effect on HF hospitalisation for values <50%.22,61 Based on this evidence, the 2022 American Heart Association/American College of Cardiology/Heart Failure Society of America guidelines give a class 2b recommendation for ARB use with the aim of reducing HF hospitalisation, especially in the lower end of the LVEF.7
Mineralocorticoid Receptor Antagonists
One large, multicentre, randomised, double-blind, placebo-controlled clinical trial and other smaller studies have tested the efficacy and safety of MRA in HFpEF. The TOPCAT trial enrolled patients aged >50 years with at least one sign or symptom of HF and VEF >45%, history of HF hospitalisation in the previous 12 months or natriuretic peptide (NP) level.22
The overall result on the primary outcome of a composite CV death and HF hospitalisation was neutral (HR 0.89; 95% CI [0.77–1.04]; p=0.14), as only HF hospitalisations were significantly reduced in the treatment arm (HR 0.83; 95% CI [0.69–0.99], p=0.04). Two clearly diverging trajectories in the occurrence of the primary outcome emerged since the first publication of the results.62 Patients enrolled with the HF hospitalisation criterion recorded a far lower rate of events than those enrolled with the NP criterion. Regional differences were also striking, with patients enrolled in Russia and Georgia (mostly with the hospitalisation criterion) showing a lower risk profile, few events and younger age. A post hoc analysis proved that the outcome was met in patients enrolled in countries other than Russia and Georgia. To make things worse, the significantly lower level of patients from Eastern Europe compared with the rest of the trial countries raises the legitimate suspicion that many participants were not taking the drug at all.63
The TOPCAT trial shaped much of our knowledge around the use of MRA in HFpEF, being the only large-scale clinical trial on clinically relevant outcomes. Smaller studies found beneficial effects of MRA on surrogate outcomes (mainly echocardiographically assessed DD parameters, reverse remodelling and NP decrease), but not on QoL, symptoms and exercise capacity.64,65
New trials are ongoing to better define the role of MRA in HFpEF. SPIRRIT-HFpEF and FINEARTS-HF (NCT04435626) will hopefully shed light on this.66
The 2022 American Heart Association/American College of Cardiology/Heart Failure Society of America guidelines give a class 2b recommendation for MRA use, with the aim of reducing HF hospitalisation, especially in the lower LVEF range.7 However, the European guidelines do not mention MRA for HFpEF.6
Antagonist Receptor Neprilysin Inhibitors
The PARAGON-HF trial randomised 4,822 patients with NYHA class II–IV, EF >45%, elevated NP and structural heart disease to valsartan alone or sacubitril/valsartan.67 The primary endpoint was a composite of total HF hospitalisation or CV death. The primary endpoint was missed for a small number of events (just seven events). Splitting the components of the outcomes, a truly neutral effect on CV mortality (HR 0.95; 95% CI [0.79–1.16]) and a modest positive effect on HF hospitalisations (rate ratio 0.85; 95% CI [0.72–1.00]) were evident. Other important secondary outcomes (NYHA class improvement, change in QoL assessed by the Kansas City Cardiomyopathy Questionnaire and renal function preservation) showed benefit from ARNI treatment compared with valsartan.
Prespecified subgroups analysis highlighted a different response to treatment in women (who benefitted most) compared with men and, as seen in the CHARM Preserved and TOPCAT trials, in the lower end of the EF spectrum, with an efficacy of the treatment up to an EF of 57%.68
Recently, the PARAGLIDE study randomised 466 patients with LVEF >40 % and a recent event of worsening heart failure to sacubitril/valsartan versus valsartan. The primary endpoint of N-terminal fraction of pro-BNP reduction was greater in the sacubitril/valsartan group (p=0.0049), and was associated with clinical benefit as reduced worsening renal failure compared with valsartan alone, despite a more symptomatic hypotension.69
A recent pool analysis of PARAGLIDE and PARAGON showed that in a population of 5,262 patients, sacubitril/valsartan versus valsartan alone significantly reduced CV death and total worsening heart failure events (p=0.027). These data encourage the use of ARNI in HF with mildly reduced or preserved LVEF, particularly in those with a LVEF below normal.70
β-blockers
β-blockers have been studied less intensively and in smaller clinical trials compared with the RAASi agents. As a consequence, their use in this population is still subject to clinical judgement and tailored care. The heterogeneity of the HFpEF population, and the various underlying mechanisms and aetiologies make the design of clinical trials even more difficult than with other agents.
The SENIORS trial enrolled HF patients across the entire spectrum of the LVEF and randomised them to nebivolol or a placebo.71 The subgroup analysis of 752 patients with LVEF >35% is the largest cohort randomised to a placebo we have to date. A smaller study, the J-DHF, tested carvedilol compared with a placebo in a smaller cohort of patients; several metanalyses put together data from randomised clinical trials and observational cohorts with or without propensity score analysis, but the overall quality of the data was low, and the results are inconsistent throughout the studies. Nonetheless, metanalyses suggest that β-blockers could reduce CV mortality or all-cause mortality without significant effect on HF hospitalisation.72–75
In all these clinical trials, β-blocker withdrawal was high due to drug intolerance. Chronotropic incompetence related to β-blockers is a well-recognised contributor to exercise intolerance commonly observed in HFpEF.76 One study reported significant improvement in functional capacity measured by peak oxygen consumption after β-blocker withdrawal in patients with HFpEF and chronotropic incompetence.77
Treating Cardiac Comorbidities in HFpEF
HFpEF is a complex syndrome characterised by non-cardiac and cardiac comorbidities, conditioning phenotypes and affecting prognosis. In this review, we focus on the latter, underlying the potential therapeutic options to improve symptoms, QoL and outcomes (Figure 2).78
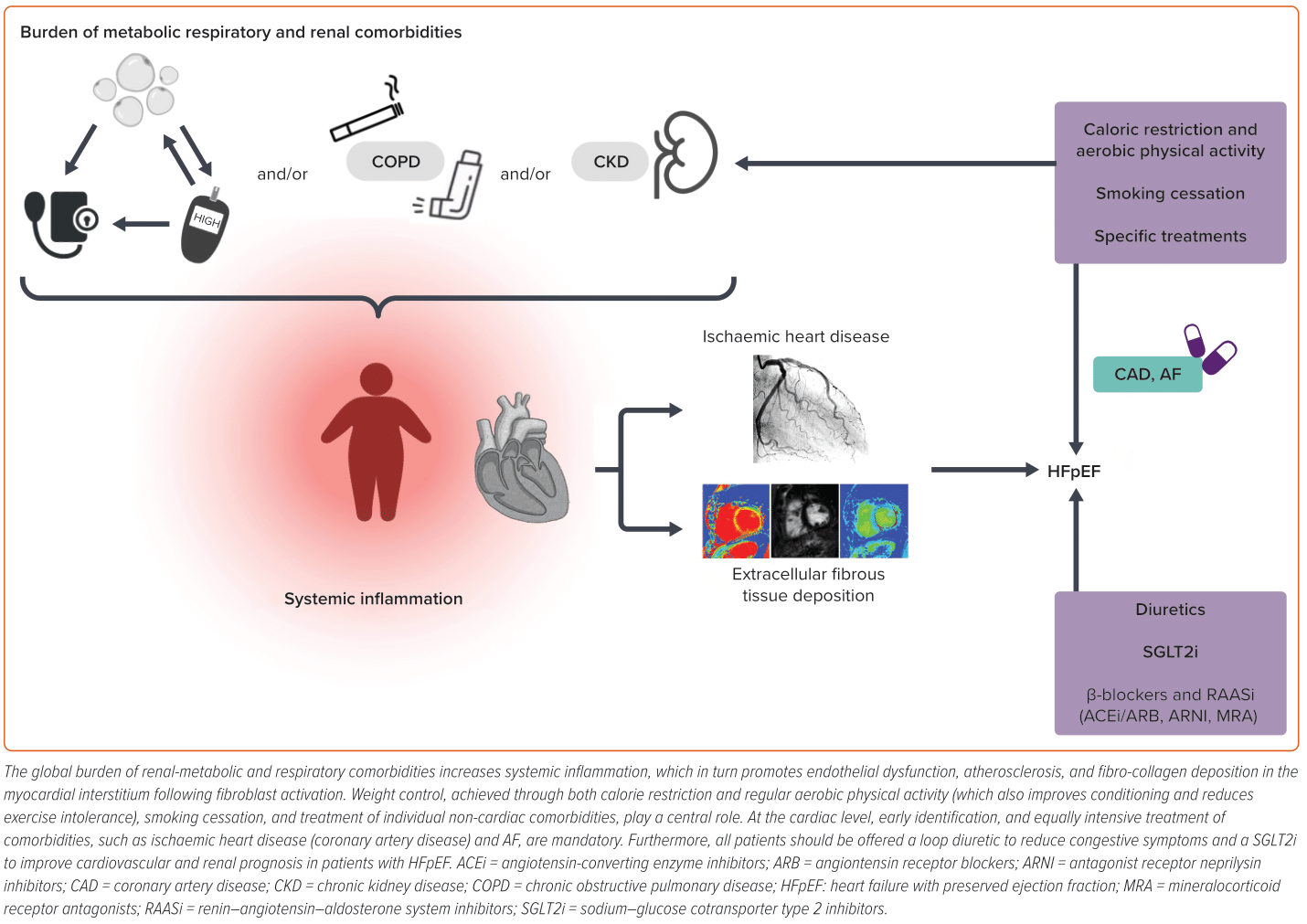
Ischaemic Heart Disease: A General Term for Several Conditions Related to HFpEF
Ischaemic heart disease and HF are strongly and tightly connected conditions.79–83 Ischaemic heart disease is a common cardiac comorbidity in HFpEF, even though prevalence varies across studies, also depending on the imaging modalities used to ascertain it and on the general arterial involvement. Ischaemic heart disease includes microvascular dysfunction (MVD), coronary artery disease or previous ‘silent’ MI.84,85
MVD contributes to the pathophysiological mechanisms of HFpEF in several ways. The pro-inflammatory state, exacerbated by the multiple comorbidities present in HFpEF, promotes oxidative stress and endothelial dysfunction. The most important mechanisms leading to MVD in HFpEF consist of microvascular inflammation, capillary rarefaction and cardiac fibrosis.86–89 The involvement of MVD in the pathophysiology of HFpEF is crucial to identify possible therapeutic targets. SGLT2s have shown beneficial effects of cardiac microvascular endothelial cells on cardiomyocyte function, and demonstrate an improvement in myocardial flow reserve in a single-centre prospective randomised clinical trial.90
Ranolazine inhibits the late sodium current and reducing intracellular calcium in cardiomyocytes and promotes ventricular relaxation by decreasing diastolic tone and microcirculatory resistance, thus improving coronary blood flow. It also acts on cardiac fibrosis; thus, it could improve the most important physiological mechanisms of MVD in this syndrome.91
New studies on antidiabetic agents, glucagon-like peptide-1 agonists, are ongoing on the obese HFpEF phenotype, and they could also have a role in chronic inflammation and MVD.26
Atrial Fibrillation
AF is a highly prevalent cardiac comorbidity in HFpEF, ranging from 32% to 65% in epidemiological studies.92,93 Despite this difference in absolute prevalence, AF is consistently reported to be more common in HFpEF than in HFrEF, and to be associated with older age. An interesting analysis of the HF Long-Term Registry of the European Society of Cardiology, evaluating both ambulatory and hospitalised HF patients, showed that after multivariable adjustment, AF significantly increased long-term mortality and hospitalisation just in the HFpEF and HFmrEF population, but not in the HFrEF population.94.
Patients with AF and HFpEF should be anticoagulated according to guidelines, keeping in mind the high incremental thromboembolic risk added by HF.95 Based on the available evidence, no firm recommendation can be drawn on rhythm versus rate control strategy preference.
Rate control is still a very popular choice among clinicians, and convincing evidence to support a routine rhythm control strategy is still missing. The two major trials on this subject and subsequent metanalysis suggest no benefit of one strategy over the other.96–98 Three drug classes are valuable options for rate control in HFpE: β-blockers, non-dihydropyridine calcium channel blockers and digoxin. Few studies have directly compared these drugs. The RATE-AF trial compared low-dose digoxin and bisoprolol in HF patients with permanent AF.99 The vast majority of the trial cohort (81%) had HFpEF. The primary endpoint (QoL at 6 months from randomisation) was not different in the two groups, and fewer adverse events were recorded in the digoxin group (p<0.001).
Diabetes and Metabolism Disorders
Diabetes, excess weight and obesity, typical features of HFpEF patients, as already documented in the above-mentioned phenotypes, cause inactivity and deconditioning, justifying the high prevalence of dyspnoea and exercise intolerance in this population. Weight loss, through caloric restriction or increased physical activity, and glycaemic control have a positive effect on prognosis and CV events. Notably, visceral and pericardial adipose tissues play a negative, pro-inflammatory role compared with subcutaneous adipose tissue, emphasising that ‘not all fat is equal’.100 Metabolically active fat, responsible for releasing inflammatory adipokines into circulation, exerts a detrimental impact on the heart and other organs, particularly the kidneys, where fibrotic degeneration leads to diastolic dysfunction and chronic kidney disease. Additionally, obesity is associated with obstructive sleep apnoea, contributing to increased right ventricular afterload, promoting functional deterioration, and eventually its failure.101
From the therapeutic perspective, glucagon-like peptide-1 agonists may represent a future therapeutic target for patients with HFpEF, acting on several pathways involved in the pathophysiology of HFpEF, including diabetes and obesity.
It has been demonstrated that the use of liraglutide is associated with reduced body weight and improved metabolic control. The LEADER study demonstrated that the rate of the first occurrence of death from CV causes, non-fatal MI or non-fatal stroke among patients with type 2 diabetes was lower with liraglutide than with a placebo.102
Moreover, in the STEP-HFpEF trial, semaglutide significantly reduced body weight, improving symptoms with a positive impact on QoL in obese HFpEF patients.26
Supplementary Table 1 shows the most important trials on RAASi, SGLT2i and glucagon-like peptide-1 agonists in HFpEF described in this review.
Devices for HFpEF
Transcatheter Interatrial Shunt Device
One of the key haemodynamic features of HFpEF is elevated left atrial and pulmonary venous pressure at rest or on exertion, resulting in dyspnoea and reduced exercise capacity. A transcatheter-implanted interatrial shunt device has been developed with the aim of opening an 8 mm large left-to-right atrial shunt and unloading left atrium. The REDUCE LAP-HF I trial, a phase II clinical trial, demonstrated a greater reduction of exercise pulmonary capillary wedge pressure in patients on active treatment compared with patients treated with a sham procedure.103 A phase III clinical trial, REDUCE LAP-HF II, was then conducted to test if the results could translate into CV death, non-fatal ischaemic stroke and HF events reduction, or change in QoL measured by the Kansas City Cardiomyopathy Questionnaire overall summary score.104 The trial enrolled 1,072 patients, of which 314 underwent an atrial shunt device procedure and 312 a sham procedure. The overall population results were neutral both for the composite primary endpoint (win ratio 1.0; [95% CI 0.8–1.2]; p=0.85) and for the single components of the outcome. The analysis of prespecified subgroups showed that in the highest tertiles of exercise pulmonary artery pressure (pulmonary pressure >70 mmHg) and right atrial volume (>29.7 ml/m2), the HF events rate was higher in the device-treated patients compared with those in the sham procedure arm, suggesting a detrimental effect of the implanted interatrial shunt device. Several clinical trials are ongoing or still have to start on the role of inter-atrial devices, and they hopefully will be able to shed light on the net clinical benefit of this kind of strategy in HFpEF (FROST-HF study, NCT05136820).105
Cardiac Contractility Modulation
Abnormal myocardial contractility, and pathological atrial and ventricular remodelling is a key feature of HFpEF. Nowadays, a new therapeutic option could be counted in this contest, cardiac contractility modulation (CCM). CCM is a new device acting through myocytes stimulation during the absolute refractory period of the action potential, leading to an increase in peak calcium concentration and inducing positive inotropism.106 CCM has been shown to improve tolerance to exercise and outcome in HFrEF patients, and growing data exist on the benefits of HFmrEf in terms of functional status and QoL.107,108 A prospective, multicentre, single-arm study (CCM-HFpEF) was designed to assess the impact of CCM in a bigger HFpEF cohort. Over a 24-week follow-up period, in a population of 47 patients in NYHA II and III with LVEF >50%, CCM caused an improvement in health status and the Kansas City Cardiomyopathy Questionnaire with no impact on safety outcomes.109 Further randomised clinical trials are needed to better define the potential impact on outcome and prognosis of this innovative device in HFmrEF and HFpEF.
Conclusion
HFpEF is a complex syndrome characterised by profound clinical and pathophysiological heterogeneity that accounts for the difficulty in identifying a unique pharmacological treatment. The identification of specific clinical phenotypes could play a role in choosing specific strategies aimed at treating specific pathophysiological alterations and comorbidities, both cardiac and non-cardiac, with the aim of improving the quantity and, above all, the quality of life of the patient as quickly as possible. Currently, SGLT2 inhibitors represent the only class of drugs with a beneficial role in the treatment of HFpEF, likely due to their ability to act across multiple common pathophysiological aspects of different disease phenotypes.
Ultimately, the goal of phenotyping is not only to better understand the complex pathophysiological mechanisms underlying HFpEF, but also to provide cardiologists with as many tools as possible to improve the quantity and, above all, the quality of life of their patients.