Imaging and Cardiomyopathy Phenotype
Cardiomyopathies (CMPs) are a group of diseases affecting the cardiac muscle, heterogeneous in terms of aetiology and clinical presentation. In 2008, a European Society of Cardiology (ESC) position statement defined CMPs as myocardial disorders “in which the heart muscle is structurally and functionally abnormal, in the absence of coronary artery disease, hypertension, valvular disease and congenital heart disease sufficient to cause the observed myocardial abnormality”.1
CMPs were grouped into specific morphological and functional phenotypes (hypertrophic, dilated, arrhythmogenic, restrictive, or other CMPs), and each phenotype was sub-classified into familial/genetic and non-familial/non-genetic forms.1 CMPs present variable expressions and symptoms, which may change over time.
CMPs may represent a challenge for cardiologists and imaging specialists, but an in-depth knowledge of aetiologies and characteristic features can lead to the correct diagnosis. The diagnostic workup of patients with suspected CMP includes clinical history and physical examination, a 12-lead ECG, specific laboratory tests, and often an endomyocardial biopsy and genetic testing. Cardiac imaging plays a pivotal role in the diagnosis of CMPs and their differentiation from mimicking conditions, such as athlete’s heart. Transthoracic echocardiography (TTE) is a safe, readily available technique that may raise the suspicion of CMP or allow a first assessment of patients with suspected CMP. Other imaging modalities, such as cardiac magnetic resonance (CMR), cardiac CT (CCT) and nuclear imaging, may help come to the correct diagnosis. In some cases, imaging findings alone are sufficient to diagnose specific disorders with no need for histological demonstration. The most notable case is amyloid transthyretin (ATTR) cardiac amyloidosis (CA), which can be diagnosed when there is an intense myocardial uptake of bone tracers on scintigraphy, and no monoclonal protein is found.2
Besides aetiological diagnosis, imaging techniques may help detect cardiac involvement in an earlier stage, characterise the remodelling pattern, perform an accurate functional assessment and risk stratification, and finally guide therapeutic decisions. TTE also offers the possibility of serial examinations over time. When possible, basic TTE examination should be integrated by speckle-tracking imaging of the left ventricle (LV), and, ideally, also of other cardiac chambers. CMR provides additional anatomical and functional information and enables tissue characterisation, which has important prognostic implications. Other techniques, such as CCT, scintigraphy or PET, may provide additional information to predict future disease trajectories and guide patient management.3 Table 1 provides an overview of the utility of different imaging modalities for the assessment of CMPs.
Overall, multimodality cardiac imaging plays an important role in clinical decision-making and helps to improve patients’ management and outcomes. In this review, we provide an overview of the current applications and some future perspectives on the use of imaging techniques in the diagnosis and treatment of patients with CMPs.
3D Echocardiography
3D echocardiography provides real-time images of the heart in motion that can be displayed at full or cropped volume, allows a more accurate study of cardiac geometry (LV and RV volumes, LV mass) and function (LV and RV ejection fraction, LV regional wall motion, dyssynchrony, and dyssynchrony index). Measurements of linear dimensions and areas can be based on 3D-guided 2D, which ensures higher accuracy than 2D measurements, or on volumetric rendered images that highly depend on image processing.7
Speckle Tracking Echocardiography
Speckle tracking echocardiography (STE) enables the calculation of motion, velocity, strain and strain rate by identifying a specific image feature, following it frame after frame, and analysing its displacement over time. Good image quality is necessary for STE.7 Global longitudinal strain (GLS) is one of the most studied parameters to detect preclinical disease, especially in DCM.17 Studies on HCM have focused on LA peak strain during the reservoir phase, corresponding to LV systole, while in ACM RV GLS can be calculated by averaging RV peak systolic longitudinal strain values from six RV segments.12,18 Conversely, RV free wall longitudinal strain is to be preferred to RV GLS in takotsubo syndrome.19 In patients with suspected CA, peak LA longitudinal strain and peak atrial contraction strain have independent diagnostic value (Figure 1).20 LV and RV strain, as well as LA enlargement, RV dilatation and RV contractile dysfunction, are important prognostic markers in DCM.7 Longitudinal myocardial function can be studied also in cardiac sarcoidosis with 2D STE or TDI-derived strain, allowing the identification of the disease at early stages, when other 2D echo features may be absent.21
Stress Echocardiography
Stress echocardiography dynamically evaluates myocardial structure and function under a stress condition induced by physical exercise (using a treadmill or a bicycle) or a pharmacological agent (inotrope or vasodilator). Images are acquired at baseline, at a low workload and at peak exercise.7 Myocardial contractile reserve, studied with stress echocardiography, helps distinguish ischaemic from non-ischaemic disease and holds prognostic importance.22,23 Moreover, a limited coronary flow and a reduced or absent contractile reserve are often present in subclinical
CMPs.24–26 Exercise echocardiography is used to quantify mitral regurgitation and to study inducible LVOTO (which is typically increased at the beginning of the recovery phase) in HCM patients.24
Cardiac Magnetic Resonance
CMR is a multi-parametric, highly reproducible, non-invasive imaging technique with a relatively high spatial, temporal and contrast resolution, which has become an essential tool for the evaluation of CMPs. Indeed, CMR allows not only the quantification of biventricular volumes, mass, wall thickness, systolic- and diastolic function, intra- and extracardiac flows, but also the detection of myocardial oedema, fibrosis, and the accumulation of other intra/extracellular substances (such as fat, iron, or amyloid), thus providing unique information for disease characterisation.27,28 It has only a few contraindications, mostly related to MR-unsafe metal implants, severe renal dysfunction (which limits the use of several gadolinium-based contrast agents), patient discomfort (claustrophobia), tachyarrhythmias or poor breath-holding ability (which might significantly degrade image quality).29
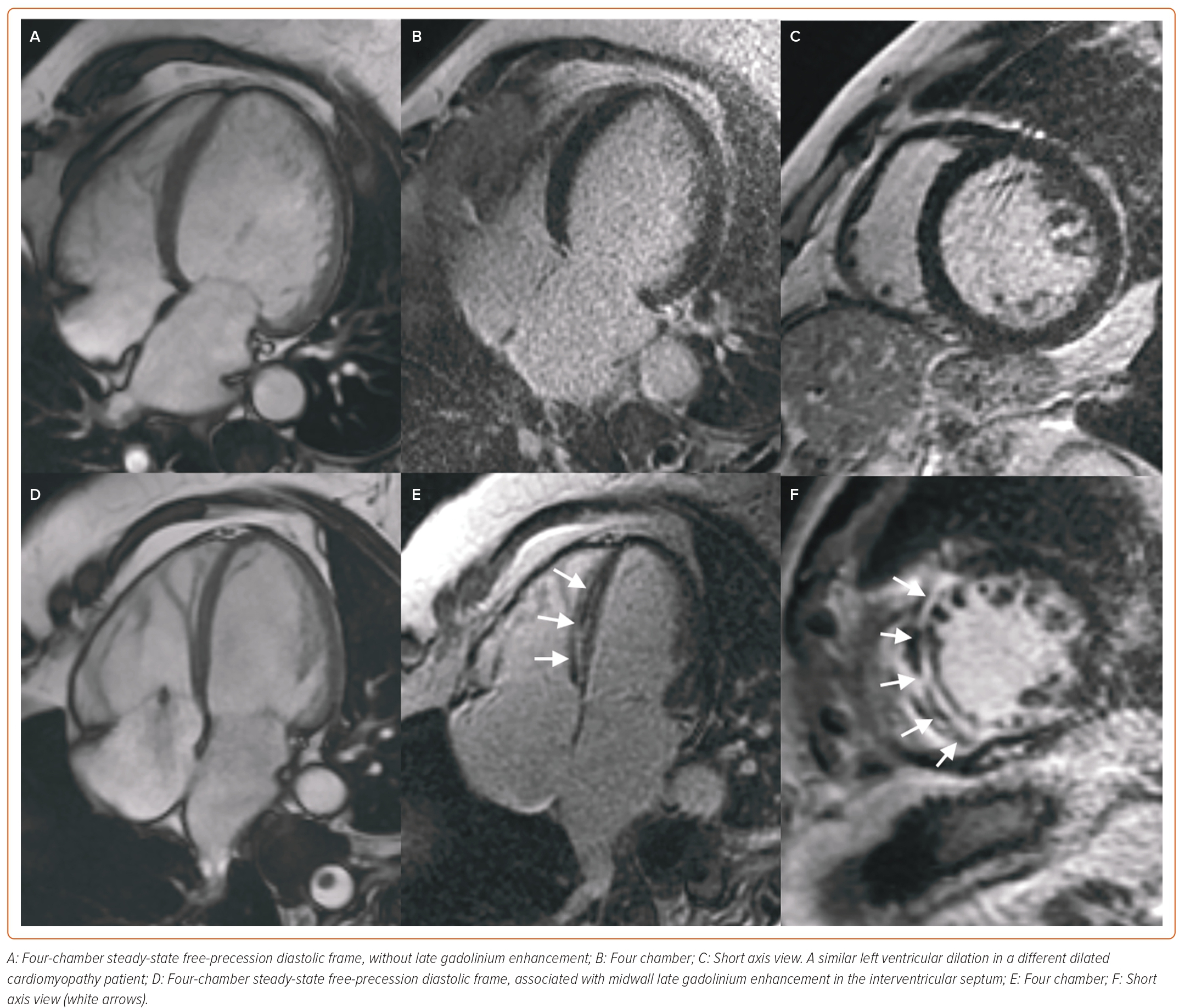
In patients with non-ischemic DCM, CMR is the gold standard technique for the quantification of biventricular volumes, mass, and EF with cine steady-state free-precession (SSFP) sequences, and for the assessment of myocardial fibrosis with late gadolinium enhancement (LGE) sequences.30 LGE can be found in about 30–50% of DCM patients, typically in a patchy, midwall or subepicardial distribution (Figure 2). Since the first study by Assomull et al. in 2006, midwall fibrosis detected by LGE has emerged as a predictor of adverse prognosis, including all-cause and cardiovascular mortality, sudden cardiac death (SCD), appropriate ICD therapy and ventricular arrhythmias (VA), independently from left ventricular ejection fraction (LVEF).31–33 Interestingly, while the relationship between LVEF and VA or SCD is weak in DCM patients, a strong, significant association has been demonstrated between LGE and VA or SCD, even in patients with LVEF >35%. Different LGE location, pattern or extent have different prognostic impacts, although further larger investigations are needed.34,35 The absence of LGE predicts the occurrence of reverse remodelling, while the presence and transmural pattern of LGE in the LV lateral wall predicts a poor response to cardiac resynchronisation therapy (CRT).36–39 Native myocardial T1 and extracellular volume (ECV) mapping track myocardial fibrosis and interstitial remodelling, and have recently emerged as independent markers of poor outcome.40–42 Recently, GLS measured by feature-tracking analysis of cine SSFP images was found to correlate better than LVEF and B-type natriuretic peptide with the composite outcome of cardiac death, heart transplantation and appropriate ICD shock.43 CMR plays a crucial role also in the detection of LV noncompaction (LVNC), whose diagnosis is particularly challenging, especially due to the overlap with other CMPs and normal LV trabeculation. Besides several geometric diagnostic criteria with limited diagnostic and prognostic utility, the most powerful prognostic parameters in LVNC are the presence of LV systolic dysfunction and LGE.44–48

In patients with HCM, CMR allows the morphological assessment of cardiac chambers, including unusual patterns of hypertrophy (lateral, apical or RV distribution), myocardial crypts, papillary muscle abnormalities, elongated mitral valve leaflets and apical aneurysms, which are not always easily visualised by echocardiography.49–52 Myocardial fibrosis detected by LGE imaging is a common finding in HCM, occurring in up to 80% of patients (Figure 3), so that only LGE extent is predictive of outcome: an LGE threshold of 10–15% of the LV mass identifies patients at high risk of VA, even in the absence of other major risk factors, and has been listed among the criteria to be considered for the selection of patients to be referred for ICD in the recently updated American Heart Association/American College of Cardiology (AHA/ACC) guidelines.53–57 Even scar heterogeneity (expressed as ‘dispersion map of LGE’), scar channels (assessed with an advanced post-processing analysis to differentiate the scar core and the border zone of LGE images), longitudinal strain, myocardial oedema (assessed through T2-weighted imaging), native T1 and ECV mapping are emerging as prognostic markers in HCM patients.58–64
CMR is of utmost importance for the differential diagnosis of hypertrophic phenocopies. In particular, CA is characterised by diffuse subendocardial-to-transmural LGE with variable biventricular and biatrial involvement, which tracks amyloid deposition and patient prognosis: while only subtle LGE areas might be present in the very early disease stages, LGE imaging can be challenging in advanced stages, due to the diffuse nature of LGE and to the equalisation of myocardial and blood pool nulling point.65 An increased native T1 demonstrated high diagnostic accuracy in patients with suspected CA, but ECV represents the best parameter to assess amyloid burden, patient outcome and response to treatment.66–71
CMR holds strong potential for diagnostic, prognosis and therapeutic monitoring in Anderson-Fabry disease (AFD), particularly because intracellular glycosphingolipids accumulation causes a shortening of native T1, while ECV is normal compared to other CMPs characterised by interstitial infiltration.72
CMR represents an essential tool for the diagnosis and risk stratification of iron overload CMPs, including genetic haemochromatosis and hereditary anaemias: myocardial iron deposits reduce both T2* and native T1 relaxation times, thus allowing an accurate non-invasive diagnosis of cardiac siderosis and treatment monitoring.73,74

ACM is a genetically determined heart muscle disease characterised by myocardial fibro-fatty replacement with variable RV and LV involvement, clinically associated with malignant ventricular arrhythmias and SCD.75 CMR has always been regarded as a tool for the non-invasive detection of major morpho-functional abnormalities (biventricular dilation, dysfunction and regional wall motion abnormalities) (Figure 4). In the recently published Padua criteria, CMR has gained further importance, with the inclusion of LGE as a major structural criterion besides endomyocardial biopsy.76 The presence of circumferential LV subepicardial LGE in short axis view (ring pattern) has been consistently reported in left-dominant forms with a specific genotype.77 Similarly to DCM, LV LGE identifies patients with a high arrhythmic risk who are candidates to ICD implantation regardless of the severity of systolic dysfunction.78 CMR can also detect myocardial fatty infiltration as hyperintense areas on T1- or proton density (PD)-weighted images, or as India ink artefacts at conventional cine-SSFP images, which nonetheless have limited sensitivity and specificity in the context of a low spatial resolution.79,80 T2-weighted images might allow the depiction of myocardial oedema in case of an ACM presentation with chest pain and troponin release, which might be encountered in paediatric
patients and carriers of DSP (desmoplakin) gene mutations with a clinical presentation similar to a recurrence of myocarditis.81,82
Nuclear Medicine
Nuclear medicine offers imaging tools to evaluate myocardial perfusion, innervation and metabolism induced by inflammatory processes as in myocarditis and cardiac sarcoidosis, or in CA. In the last decade, the use of specific single-photon emission CT (SPECT) radiopharmaceuticals has allowed the identification of the presence of ATTR-CA, while the use of new PET radiopharmaceuticals could help to achieve an early diagnosis of immunoglobulin light-chain (AL) CA without the need for biopsy confirmation.
Perfusion and Innervation SPECT Tracers
SPECT with tracers such as 201Tl, sestamibi or tetrofosmin labelled with 99mTc allows the exclusion of the presence of ischaemia, particularly in patients with HCM who present with chest pain or who have LV systolic dysfunction. Using dedicated software, it is possible to estimate the systolic and diastolic function of the LV from a myocardial perfusion study.83,84 In cardiac sarcoidosis, the assessment of LV contraction dyssynchrony by myocardial perfusion-gated SPECT can be useful for prognostic stratification; furthermore, the assessment of perfusion recovery after corticosteroid therapy seems to be associated with a lower risk of major adverse cardiac events.85,86
SPECT with 123I-labeled metaiodobenzylguanidine (MIBG) allows the evaluation of the integrity of myocardial adrenergic innervation. In AFD, the extent of impairment of adrenergic innervation correlates with the degree of fibrosis, but autonomic dysfunction appears to precede myocardial fibrosis, so the innervation scintigraphy could allow an earlier diagnosis of this disease.87 A reduction in the density of adrenergic endings has also been reported in CA, probably as a consequence of the toxic effect of the amyloid fibrils.88,89 The use of 123I-MIBG for the evaluation of adrenergic damage has also been described in patients with cardiac sarcoidosis, but it is not routinely adopted.90,91
PET Tracers Assessing Myocardial Inflammation
18F-labelled fluorodeoxyglucose (FDG) is the main radiopharmaceutical used in PET. Although its main use is oncological, it is not tumour-specific, and it allows the visualisation of areas of leukocyte infiltration during inflammatory processes. The degree of glucose uptake in the vital myocardium correlates directly with the circulating levels of insulin and inversely with the level of circulating free fatty acids, while the degree of glucose metabolism of the inflammatory tissue is relatively independent of insulin. For this reason, the use of 18F-FDG for the study of myocarditis requires an adequate dietary preparation that suppresses the myocardial glucose uptake.92–94 PET with 18F-FDG can be used to characterise the inflammatory response after acute myocardial infarction, to assess the extent of the disease and the response to therapy in the case of myocarditis and in sarcoidosis.95–98 Lymphocytes involved in the granulomatous process of sarcoidosis express membrane receptors for somatostatin; new PET radiopharmaceuticals with an affinity for somatostatin receptors, such as the peptides DOTATOC, DOTATATE and DOTANOC labelled with 68Ga, have been shown to have a greater diagnostic accuracy than 18F-FDG for cardiac sarcoidosis.99
Nuclear Medicine in Cardiac Amyloidosis
Some SPECT tracers originally developed to study bone metabolism and labelled with 99mTc have been successfully used to identify ATTR-CA. 99mTc-3,3-diphosphono-1,2-propanodicarboxylic acid (DPD) cardiac uptake in patients with ATTR-CA has been known since the 1970s; thanks to the evidence collected in the last 15 years, bone tracer scintigraphy has been a fundamental tool in the diagnostic path of patients with suspected ATTR-CA.100 18F-NaF is a PET radiopharmaceutical for bone imaging that also accumulates in myocardial ATTR deposits.101,102 However, a lower sensitivity of PET compared to scintigraphy has been reported.103,104 In the last decade, PET radiopharmaceuticals developed for brain amyloid substance have shown binding affinity also for myocardial amyloid, both in the context of a systemic amyloidosis with cardiac involvement and in the case of isolated CA.105–110 The most promising of these tracers is 18F-florbetaben, which has proven able not only to identify CA, but also to discriminate between AL-CA and other mimicking conditions and to evaluate the response to therapy (Figure 5).111,112
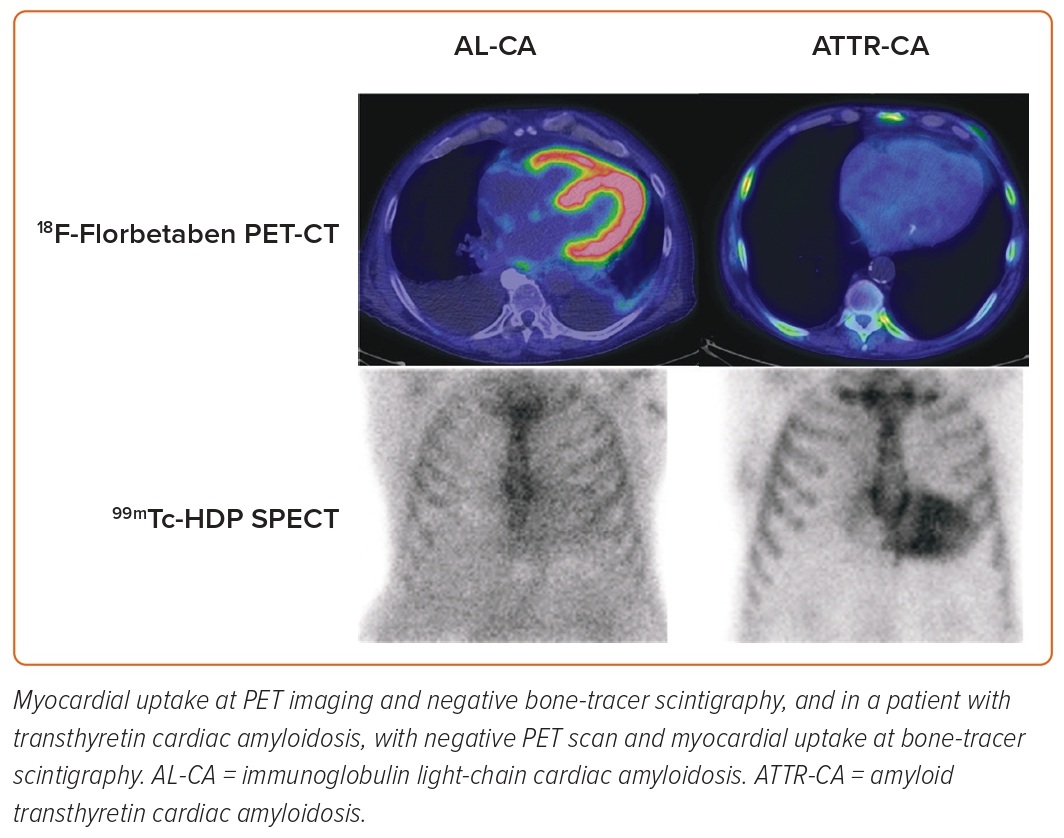
Cardiac CT
The latest generation of CCT equipment, with the implementation of temporal resolution, the use of lower kV values, high volume coverage, iterative reconstruction algorithms and low radiation exposure, allows the assessment of ventricular and atrial size, morphology, function, density,
first pass perfusion defects and delayed-enhancement pattern in sub-millimetric multiplanar reconstructions with a short acquisition time.113 In the setting of CMPs, pre-contrast CCT can easily depict myocardial areas with adipose density as well as calcifications. After contrast enhancement, in addition to providing information on the causes of ischaemia and on the risk of major cardiac events, CCT can qualitatively and quantitatively assess myocardial perfusion and the presence of myocardial muscle alterations, discriminating between ischaemic and non-ischemic aetiology, disclosing myocardial phenotype, the presence of fat, as well as complications such as intracavitary thrombosis.114,115 CCT may therefore be considered as an option in patients with contraindications to CMR or when coronary artery disease must be ruled out.116 Next challenges will concern the evaluation of myocardial metabolism with CCT photon counting and the assessment of myocardial oedema and fibrosis.117,118
Multimodality Imaging and Integration with Circulating Biomarkers
The integration of different imaging techniques may help overcome the different limitations and weaknesses of single techniques.17,119 For example, echocardiographic findings may be non-specific, therefore, have a limited role in identifying the underlying aetiology.119 CMR is generally performed after echocardiography and has a primary role in assessing the aetiology of CMPs.120 CMR findings, namely LGE and parametric imaging (T1 and T2 values), allow an accurate characterisation of myocardial tissue in terms of fibrosis, scar, inflammation, oedema, or infiltration.119,120 CCT is highly valuable to exclude significant epicardial coronary artery disease in DCM.17 Additionally, the good spatial resolution and ease of navigation make cardiac CCT an option in the presence of suboptimal echocardiographic findings and contraindications to CMR.17
The integration of imaging with circulating biomarkers allows an even more accurate characterisation of the different types of CMPs.121 For example, although the role of nuclear imaging is generally limited in the evaluation of patients with suspected CMPs, ATTR-CA is diagnosed with a 100% specificity in the presence of a positive bone scintigraphy combined with the absence of a monoclonal protein or abnormal light chains in blood samples.122 In addition, specific imaging markers provide additional information to biomarkers and refine risk prediction in AL-CA.123
Further research is required to integrate imaging findings from different techniques with clinical data and circulating biomarkers to create a multifactorial algorithm for the diagnosis and prognostic assessment, as well as for the individualisation of therapy in each specific CMP.119
Perspectives
The growing body of knowledge on the pathophysiology and natural history of CMPs, and the availability of disease-modifying therapies for some conditions have made early diagnosis more crucial than ever. However, some CMPs may show milder phenotypes in early disease stages, which can overlap between different diseases or even physiological conditions. Emblematic cases are represented by DCM versus ACM with prevalent LV involvement, or HCM versus athlete’s heart.17,124 The integration of additional information from different imaging modalities can maximise the diagnostic performance.17 For inherited CMPs with adolescent-onset, such as HCM and some forms of ACM, early diagnosis presents additional challenges, particularly for family screening. First, the diagnostic criteria have been mostly validated on adult subjects with well-differentiated phenotypes, therefore, their diagnostic performance at a younger age may be lower.125 Second, echocardiography is the preferred tool for screening, but is unable to identify subtler structural abnormalities, so it currently remains to be defined which methods and timing are the most appropriate for family screening of inherited CMPs. For example, the 2014 ESC guidelines on the diagnosis and management of HCM recommend clinical evaluation with ECG and echocardiography and long-term follow-up in first-degree relatives who have the same defined pathological mutation as the proband; when no definite genetic mutation is identified in the proband, ECG and echocardiography should be considered in first-degree adult relatives and repeated every 2–5 years (or 6–12 months if nondiagnostic abnormalities are present).126 Similar recommendations are provided by the 2020 AHA/ACC Guidelines, which also mention the possibility of employing CMR for family screening when echocardiography is inconclusive.57 As for ACM, the 2019 Heart Rhythm Society (HRS) expert consensus statement recommends clinical evaluation by ECG and cardiac imaging every 1–3 years starting at 10–12 years of age in first-degree relatives. However, the precise imaging technique to be employed is not specified.127
Another future perspective is the validation of new diagnostic methods and their integration into the diagnostic flow charts of CMPs. CMR mapping techniques have proven to be very reliable in supporting the diagnosis of CA and predicting prognosis, yet CMR currently has a secondary role in the diagnostic flow chart of CA proposed by the ESC.2 In many cases of suspected CMP, endomyocardial biopsy is still crucial.128 However, in the near future, new imaging tools might limit the need for endomyocardial biopsy in specific cases. For example, recent studies have shown that delayed cardiac uptake of 18F-florbetaben on PET/CT imaging is able to accurately discriminate AL-CA from either ATTR-CA or other phenocopies.111
Besides diagnosis, imaging tools can be used to highlight collateral structural abnormalities of some CMPs that might have clinical relevance. For example, it has been demonstrated that some forms of ACM, such as those caused by DSP mutations, can have a clinical course that passes through “hot phases” characterised by myocardial inflammation and the release of biomarkers of myocardial damage, which sometimes are misdiagnosed as acute myocarditis.81 These phases hold prognostic significance and can be identified both by CMR as the presence of oedema T2-weighted sequences and by 18F-FDG PET.124,129 Myocardial oedema detected through CMR can also be a hallmark of cardiac remodelling in HCM, and is associated with more advanced disease and increased arrhythmic load.61
Finally, the role of advanced imaging modalities in monitoring the response to therapy remains to be defined. One study on patients with ATTR-CA has demonstrated that serial ECV quantification by CMR can be used to monitor the effectiveness of reducing the cardiac amyloid burden by patisiran, a small interfering RNA able to knock down the expression of transthyretin in the liver.71 Similarly, it has been recently demonstrated that ECV quantification by CMR can be used to monitor cardiac amyloid regression following chemotherapy in AL amyloidosis. Notably, a deep haematologic response is not sufficient per se to grant cardiac amyloid regression, while changes in ECV over time independently predict prognosis, making it a unique marker of treatment response.130
Conclusion
Recent advances in cardiac imaging and its increased accessibility allow a precise assessment of ventricular dimension and function, as well as a non-invasive tissue characterisation of the myocardium. However, the growing knowledge from imaging studies should always be interpreted based on all clinical elements (i.e. clinical assessment, electrocardiographic findings, biomarkers, genetic and functional assessment). While echocardiography remains the first-line imaging tool for the management of patients with CMP and can be implemented with advanced analyses (e.g. STE, stress echocardiography, contrast-enhanced echocardiography), CMR represents the gold standard technique for myocardial anatomy and tissue characterisation, including novel tools (e.g. T1/T2/T2*/ECV mapping, quantitative perfusion, diffusion tension imaging and feature tracking). An integrated clinical and imaging approach seems essential to characterise the CMP phenotype and identify the underlying aetiologies, to predict disease prognosis and to ensure a tailored therapeutic management.
Comments