Heart failure (HF) with preserved ejection fraction (HFpEF) is defined as ‘a clinical syndrome characterised by prior or current symptoms and/or signs secondary to a cardiac structural or functional abnormality, corroborated by the presence of elevated natriuretic peptides (NPs) levels or objective evidence of cardiogenic pulmonary or systemic congestion’ in the presence of a left ventricular (LV) ejection fraction (LVEF) ≥50%.1
While the prevalence of HF with reduced ejection fraction (HFrEF) (LVEF ≤40%) is stable or decreasing, that of HFpEF is rising worldwide, probably due to various factors, including the increased awareness of clinicians in recognising this HF phenotype; the ageing population; and the increasing number of patients with HF and improved LVEF (i.e. patients with a prior diagnosis of HFrEF whose LVEF improves over time mostly because of guideline-directed medical therapy).2,3 Compared with patients with other types of LVEF, those with HFpEF have similar rehospitalisation and mortality risks, albeit with a higher proportion of non-cardiac causes, which highlights the role of comorbidities.2–5
Therapies targeting the neurohormonal systems, such as β-blockers, angiotensin-converting enzyme inhibitors/angiotensin receptor blockers (ACEIs/ARBs), mineralocorticoid receptor antagonists (MRAs) and angiotensin receptor-neprilysin inhibitors (ARNIs) are the mainstay of treatment for HFrEF and HF with mildly reduced ejection fraction (EF).
Moreover, it has been observed that, although to a lesser extent than in patients with HFrEF, those with HFpEF show a significant activation of neurohormonal systems, further supporting a potential benefit from their modulation.6 However, in the last two decades, various randomised clinical trials (RCTs) have failed to demonstrate a significant prognostic benefit of neurohormonal modulation therapies in HFpEF.7 The phenotypical and pathophysiological heterogeneity of HFpEF, together with flaws in study design, might partly explain such disappointing results.8 Nonetheless, subgroup and post-hoc analyses of RCTs suggest that some subsets of HFpEF patients (e.g. those with LVEF in the lower range of normality) might derive a benefit from those therapies.7
This review explains the pathophysiological rationale of neurohormonal modulation in HFpEF, the results of clinical trials and guideline recommendations as well as future perspectives on optimising the use of neurohormonal modulation therapies in this clinical scenario.
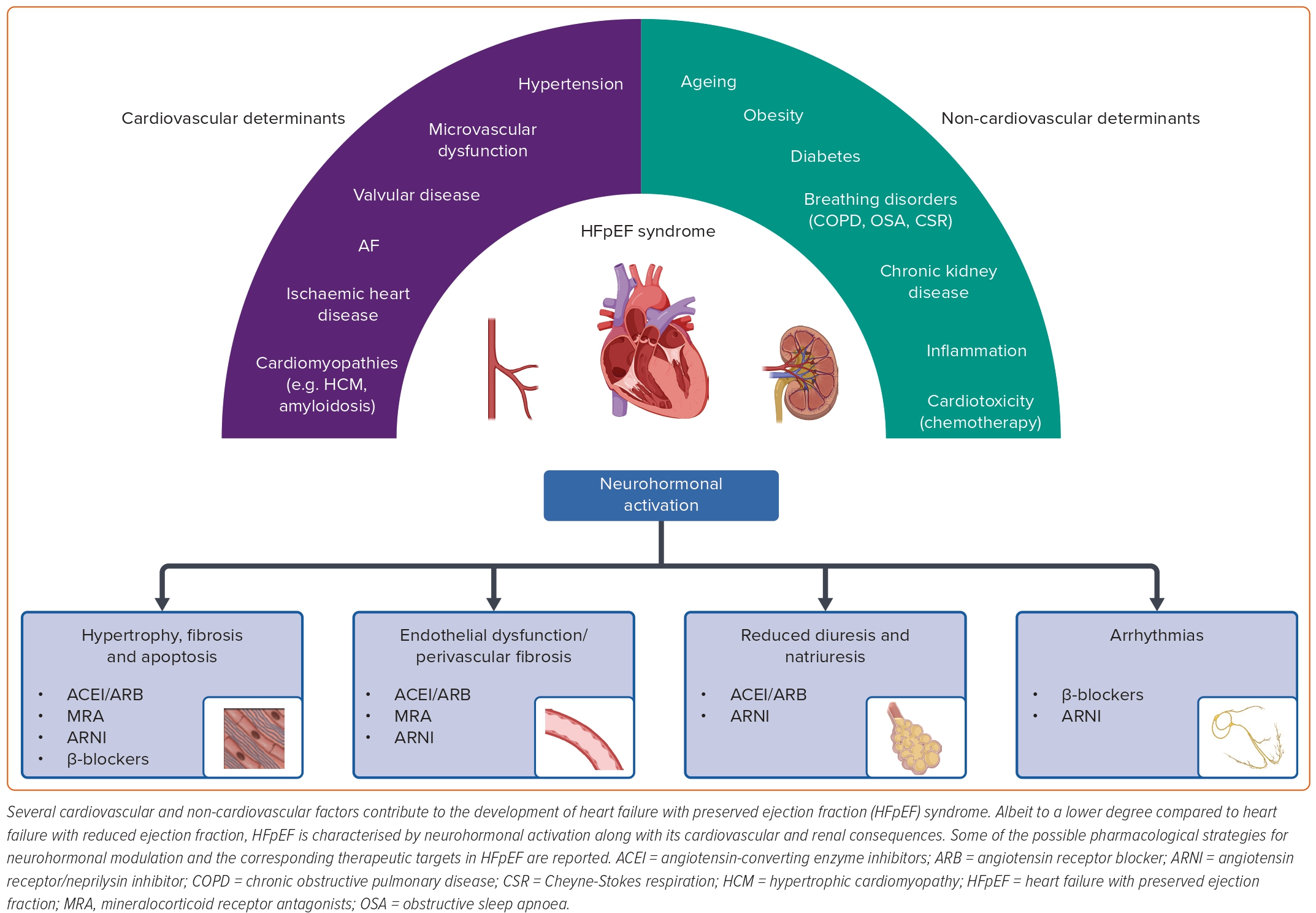
Pathophysiology of HFpEF
HFpEF derives from the interaction of various cardiac and extracardiac conditions, resulting in a heterogeneous phenotypic spectrum (Figure 1).2
At the cardiac level, increased LV filling pressures are a landmark finding in HFpEF patients, being responsible for HF signs and symptoms.9 Although diastolic dysfunction secondary to increased LV stiffness and impaired relaxation usually plays a major role, subtle systolic dysfunction can also be found in many patients through strain analysis.10
Historically, systemic hypertension has been considered the main cause of diastolic dysfunction in HFpEF, being associated with LV concentric hypertrophy and increased myocardial stiffness.11 Nevertheless, growing evidence suggests that microvascular dysfunction and cardiomyocyte metabolic/energetic inefficiency may also play a role.12,13
Finally, infiltrative disorders (e.g. cardiac amyloidosis) or other determinants of myocardial damage (e.g. related to valvular, metabolic, toxic and inflammatory conditions) can cause diastolic dysfunction in specific patient subsets.14–17 Left atrial enlargement is common in HFpEF patients, and has long been considered a direct consequence of increased LV filling pressures.18 However, as recently disclosed, left atrial dysfunction is often observed in HFpEF from an early stage, and directly contributes to disease progression.18 Similarly, post-capillary pulmonary hypertension and right ventricular dysfunction can be observed in some HFpEF patients.19,20
Compared with HFrEF, extracardiac comorbidities seem to be a major determinant in HFpEF pathophysiology.5 For example, obesity, diabetes and chronic kidney disease are highly prevalent in patients with HFpEF, promoting cardiovascular damage by activating proinflammatory and profibrotic cascades.21–23
Similarly, respiratory disorders (e.g. chronic pulmonary obstructive disease, obstructive sleep apnoeas and Cheyne-Stokes respiration) may trigger inflammatory and oxidant pathways as well as autonomic imbalance and pulmonary hypertension.23,24
Rationale for Neurohormonal Modulation in HFpEF
Both cardiovascular (e.g. hypertension, microvascular disease and cardiomyopathies) and non-cardiovascular (e.g. diabetes, renal disease and respiratory disorders) determinants of HFpEF are associated with increased activity of the renin-angiotensin-aldosterone system (RAAS) and autonomic imbalance, characterised by an enhanced sympathetic drive and vagal withdrawal. Nevertheless, compared with HFrEF, the pathophysiological role and clinical implications of neurohormonal activation have been poorly investigated in HFpEF patients.2
The degree of neurohormonal activation, as expressed by circulating biomarkers (namely plasma renin activity, aldosterone, catecholamines and N-terminal pro-B-type NP (NT-proBNP), has recently been evaluated in a well-treated HF cohort, including 189 patients for each LVEF class.6 A significant subset of HFpEF patients (67%) had elevated concentrations of at least one biomarker, while 10% of patients showed increased values in all of them (plasma renin activity, aldosterone and norepinephrine), after adjustment for the underlying HF therapy.6
The prognostic significance of RAAS activation was tested in different observational studies. Among 873 HFpEF patients, aldosterone concentrations were associated with LV concentric remodelling and independently predicted the risk of all-cause mortality and HF hospitalisation. Median aldosterone concentrations in patients with and without events were 124.22 pmol/l (48.62–256.20) and 96.33 pmol/l (37.33–215.76), respectively, (p=0.023).25 Similarly, increased plasma renin activity was identified as an independent predictor of all-cause death among 150 HFpEF patients, regardless of RAAS antagonist therapy.26 Although the specific mechanisms relating RAAS activation to poor prognosis in HFpEF have not been specifically investigated in such works, the well-known contributions of the RAAS to cardiovascular stiffness and fibrosis may be implicated here.22
Considering the pivotal pathophysiological role of the sympathovagal imbalance in HFrEF and the prognostic implications of this, various studies have investigated the possible contribution of autonomic dysregulation to the HFpEF syndrome, but have yielded contradictory results.27
Although the evaluation of heart rate variability and baroreflex sensitivity, mirroring vagal activity, was often hampered by the presence of atrial arrhythmias, patients with HFpEF seemed to show an intermediate phenotype between that of HFrEF and healthy controls.27,28 Similarly, sympathetic nervous system (SNS) activation, as estimated by norepinephrine concentrations or microneurography, increases from healthy controls to patients with HFpEF to HFrEF.27
Such observations were further confirmed in a study in which cardiac norepinephrine spillover from the heart (measured at the coronary sinus) was significantly higher in HFpEF patients than in healthy controls, and was independently correlated with wedge pressure.29 Furthermore, among 278 HFpEF patients, plasma norepinephrine concentration also retained independent prognostic significance.30
The possible determinants of sympathovagal imbalance in HFpEF are still to be clarified. Whereas age, sex and cardiac and extracardiac comorbidities may act as possible confounders, the contribution of visceral feedbacks modulating autonomic function that are often dysregulated in HFrEF (e.g. baroreflex, chemoreflex and ergoreflex) is yet to be evaluated in HFpEF patients.31,32
On the other hand, the importance of increased filling pressure and left atrial enlargement/dysfunction in HFpEF may mean cardiopulmonary reflexes have a potential role in these patients.27
Neurohormonal Modulation in HFpEF: Pharmacological Therapies
Drugs blunting RAAS and SNS activation and improving NP activity target key pathophysiological mechanisms of HFrEF and have greatly improved the prognosis for patients with this condition. Based on the evidence of neurohormonal activation in HFpEF, targeted therapies have also been tested in HFpEF (Supplementary Material Table 1).
β-blockers
β-blockers slow the heart rate, reduce myocardial contractility and increase the duration of diastolic filling. No phase II/III RCT specifically tested β-blockers in HFpEF, hence evidence of their use in this clinical setting comes mainly from observational studies and subgroup/post-hoc analyses of RCTs.
A propensity score-matched cohort study on β-blocker use among HFpEF patients from the Swedish Heart Failure Registry showed that β-blocker therapy reduced all-cause mortality but not the composite of all-cause death or HF hospitalisations.33
In a subgroup analysis of the SENIORS trial, in which randomised patients aged ≥70 years with an HF hospitalisation within the previous year or an LVEF ≤35% to nebivolol or placebo, treatment with nebivolol significantly reduced the primary composite outcome of all-cause death or cardiovascular hospitalisation regardless of LVEF class (≤35% versus >35%; p for interaction=0.42).34,35 However, the broad definition of preserved EF (namely LVEF >35%) adopted in this trial does not allow a definite conclusion to be drawn on the eventual beneficial role of nebivolol in HFpEF. Moreover, a post-hoc analysis of this trial showed that, unlike in the LVEF ≤35% subgroup, nebivolol did not improve systolic or diastolic parameters in patients with LVEF >35%.36
Notably, in a subgroup analysis of the CIBIS-ELD trial, which assessed the tolerability and clinical effects of bisoprolol versus carvedilol in HF patients aged >65 years, HFpEF patients (LVEF >45%; n=250; 29%) showed higher rates of β-blocker dose-escalation delays and treatment-related side-effects and less improvement in New York Heart Association (NYHA) class than HFrEF patients (LVEF ≤45%).37
Additionally, a post hoc analysis of the TOPCAT trial showed that patients with LVEF ≥50% treated with β-blockers at baseline had a higher risk of HF hospitalisations but not cardiovascular mortality than those not receiving β-blockers.38
Although β-blockers might limit some negative effects of SNS activation in HFpEF, some authors have suggested that their beneficial effects are offset by their negative action on the chronotropic response. Indeed, chronotropic incompetence appears to be a major driver of exercise functional capacity limitation in HFpEF.39 The recently published PRESERVE-HR study showed that β-blocker withdrawal improved peak VO2 and peak VO2% in HFpEF patients with chronotropic incompetence (defined as a chronotropic index of <0.62).39
Taken together, these data suggest that β-blocker treatment might not be suitable for all patients with HFpEF and requires re-evaluation in appropriately designed, large RCTs.
Angiotensin-Converting Enzyme Inhibitors
RAAS activation promotes myocardial hypertrophy and fibrosis, which are two key aspects of HFpEF pathophysiology, providing a rationale for testing RAAS antagonists in HFpEF.
The ACEI perindopril has been tested in the phase III RCT PEP-CHF.40 This study randomised patients aged ≥70 years with LV wall motion index ≥1.4 (roughly equivalent to an LVEF ≥40%), evidence of diastolic dysfunction, symptomatic HF treated with diuretics and hospitalisation for cardiovascular reasons within 6 months to either perindopril 4 mg daily or placebo. Treatment with perindopril did not result in a lower primary composite endpoint of all-cause mortality or HF hospitalisation at 1-year follow-up (p=0.055). Nonetheless, enrolment and event rates were lower than expected, but the study was underpowered so could not detect a statistically significant difference in the primary endpoint between the two groups.
When the individual components of the primary endpoints were assessed, perindopril was found to be effective in preventing HF hospitalisations (HR 0.63; 95% CI [0.41–0.97]; p=0.033). Improvements in NYHA class (p<0.030) and 6 minute walking distance (p=0.011) were also observed in the perindopril group. According to subgroup analysis, younger patients (≤75 years) and those with a previous history of hypertension or MI received a greater benefit from perindopril.40
Angiotensin Receptor Blockers
The CHARM-Preserved trial enrolled patients with LVEF >40%, NYHA class II–IV and a history of hospitalisation for cardiac reasons to receive either candesartan 32 mg daily or placebo. Treatment with candesartan almost met statistical significance for the primary composite endpoint of cardiovascular death or HF hospitalisation (HR 0.86; 95% CI [0.74–1.00]; p=0.051).
The number of cardiovascular deaths was equal in the two groups (170 versus 170), but fewer patients in the candesartan group were hospitalised for HF (230 versus 279; p=0.017).41 A post-hoc analysis showed that candesartan reduced the rate of recurrent HF hospitalisations to a greater extent than was evident from the assessment of first hospitalisations alone.42
Another post-hoc analysis demonstrated that the rates of the primary outcome declined with increasing LVEF. Specifically, candesartan significantly reduced the primary outcome in patients with LVEF up to 50%, and recurrent HF hospitalisations in patients with LVEF up to 60%.43
In the I-PRESERVE trial, patients aged ≥60 years with LVEF ≥45%, NYHA class III–IV or NYHA class II with HF hospitalisation within the previous 6 months were randomly assigned to receive either irbesartan 300 mg daily or placebo. After a median follow-up of 49.5 months, no significant differences were observed concerning the composite outcome of all-cause mortality or hospitalisation for a cardiovascular cause (HF, MI, unstable angina, arrhythmia or stroke).44
These results in part conflict with those from the CHARM-Preserved trial, although several factors may explain this divergence: a different definition of HFpEF (LVEF >40% versus ≥45%); a different primary endpoint, with that of I-PRESERVE including outcomes less sensitive to the effect of treatment with ARBs, such as all-cause death; a high percentage of patients concomitantly taking another RAAS inhibitor (nearly 40% received an ACEI and nearly 29% a MRA) in both study arms of the I-PRESERVE trial possibly reducing the additive benefit from irbesartan; and the presence of some imbalances in baseline variables that might have affected outcomes and/or the response to irbesartan not being adjusted for in the I-PRESERVE trial.45
In a post-hoc analysis of the I-PRESERVE trial, after adjusting for key prognostic baseline covariates such as age, sex, medical history, physiological and laboratory variables, treatment with irbesartan resulted in a HR of 0.89 (95% CI [0.80–0.99]; p=0.033) for the primary composite outcome. Similar findings were observed for the composite of cardiovascular death or HF hospitalisation.45
Another post-hoc analysis from the I-PRESERVE trial showed that patients with baseline NT-proBNP values above the median of 339 ng/l had an increased risk of cardiovascular hospitalisations (p=0.001), all-cause mortality (p=0.001) and a composite of HF events, including death due to worsening HF or sudden death or hospitalisation because of worsening HF (p=0.001). Treatment with irbesartan was associated with improved outcomes in patients with NT-proBNP values below but not above the median, even after adjusting for 20 baseline covariates.46 This suggests that the effect of RAAS antagonism in HFpEF may be more relevant in early, lower-risk stages of the disease.
Mineralocorticoid Receptor Antagonists
In the Aldo-DHF trial, the investigators randomised patients aged ≥50 years with LVEF ≥50%, NYHA class II–III, peak VO2<25 ml/min/kg, and echocardiographic evidence of diastolic dysfunction or AF to either spironolactone 25 mg daily or placebo. Treatment with spironolactone for 12 months improved LV diastolic function (assessed through a change in E/e’ ratio) and significantly reduced LV mass index and NT-proBNP, but did not affect maximal exercise capacity (assessed through a change in peak VO2), patient symptoms or quality of life.47
Several subsequent post-hoc analyses tried to identify the specific subsets of HFpEF patients who received the greatest benefit from spironolactone treatment. Ravassa et al. measured serum levels of biomarkers associated with myocardial collagen deposition (carboxy-terminal propeptide of procollagen type I) and cross-linking (ratio of serum carboxy-terminal telopeptide of collagen type I to serum matrix metalloproteinase-1) in 381 patients from the Aldo-DHF trial, finding that a biochemical phenotype of high collagen cross-linking identified patients resistant to the beneficial effects of spironolactone on diastolic dysfunction.48
Another study reported that, among patients receiving a placebo, carriers of the rs5522 G allele of the NR3C2 gene, which encodes for spironolactone’s target protein, showed a greater increase in E/e’ ratio over 12 months compared to non-carriers. Conversely, treatment with spironolactone seemed to attenuate the progression of diastolic dysfunction associated with the NR3C2 rs5522 G allele.49
A further study aimed to identify a biomarker profile associated with spironolactone effect in HFpEF. Among 92 biomarkers tested, 13 proteins including renin, growth hormone, adrenomedullin and inflammatory peptides (e.g. tumour necrosis factor receptor superfamily member 11A, interleukin-18 and interleukin-4 receptor subunit alpha) showed a significantly different expression change between spironolactone and placebo from baseline to 12-month follow-up.50
Following the positive results of the Aldo-DHF trial, the TOPCAT trial tested spironolactone (up to 45 mg daily) versus placebo in 3,445 patients from six countries (the US, Argentina, Brazil, Canada, Georgia and Russia) with an LVEF ≥45% and clinically overt HF (defined as the presence of at least one sign and at least one symptom of HF plus a history of HF hospitalisation within 12 months or elevated NP levels). Over a mean follow-up of 3.3 years, treatment with spironolactone did not significantly reduce the incidence of the primary composite outcome of death from cardiovascular causes, aborted cardiac arrest or hospitalisation for the management of HF (HR 0.89; 95% CI [0.77–1.04]; p=0.14).51
However, issues regarding patient enrolment in certain locations (namely Russia and Georgia) were raised. In particular, patients enrolled on the basis of the hospitalisation criterion had a lower event rate than those enrolled on the basis of the NP criterion and were younger and had fewer coexisting conditions.
The majority of patients from Russia and Georgia were enrolled according to the hospitalisation criterion and were possibly at lower risk.52 In a post-hoc analysis, treatment with spironolactone met the primary endpoint when the patients from these countries were excluded.53
Angiotensin Receptor–Neprilysin Inhibitors
Sacubitril/valsartan is a first-in-class angiotensin receptor–neprilysin inhibitor with proven prognostic benefit in HFrEF. Sacubitril/valsartan combines the positive effects of RAAS antagonism with those of NP enhancement, making it an actual neurohormonal modulator.
The PARAMOUNT trial randomised 149 patients with LVEF ≥45%, symptomatic HF (NYHA class II–III), and NT-proBNP >400 ng/l to either sacubitril/valsartan (target dose of 97/103 mg twice daily) or valsartan (target dose 160 mg twice daily). Treatment with sacubitril/valsartan produced a greater reduction in NT-proBNP from baseline to week 12 compared to valsartan (primary outcome; p=0.005). However, at 36 weeks, the difference between the two treatment groups was no longer significant. Nonetheless, at 36 weeks, patients taking sacubitril/valsartan showed a greater reduction in left atrial size and a significant improvement in NYHA class compared to those taking valsartan, although overall quality of life assessed through the Kansas City Cardiomyopathy Questionnaire (KCCQ) did not differ between the two arms.54
After PARAMOUNT, the phase III PARGON-HF trial was intended to demonstrate a beneficial effect of sacubitril/valsartan over valsartan in terms of cardiovascular events. All patients had symptomatic chronic HF (NYHA class II–IV), LVEF ≥45% and NT-proBNP levels >300 ng/l (or >900 ng/l if in AF at screening). If a hospitalisation for HF had occurred in the previous 9 months, NT-proBNP cut-offs were 200 ng/l and 600 ng/l for sinus rhythm and AF, respectively. Patients were randomised 1:1 to sacubitril/valsartan or valsartan, with the same target doses as in the PARAMOUNT trial. At a median follow-up of 35 months, the study did not demonstrate a significant reduction in the composite primary endpoint of total HF hospitalisations and cardiovascular death between the two groups (p=0.06).
However, subgroup analysis showed that sacubitril/valsartan reduced the primary endpoint in patients with an LVEF below the median (57%), those with a reduced baseline estimated glomerular filtration rate (<60 ml/min/1.73 m2) and women.55 A study pooling data from the PARADIGM-HF and the PARAGON-HF trials confirmed these results, showing that the prognostic advantage of treatment with sacubitril/valsartan over ACEI/ARB persisted in patients with LVEF up to values just below the normal range.56
In the PARAGON-HF trial, sacubitril/valsartan demonstrated a greater improvement in NYHA class and a lower decline in KCCQ Clinical Summary Score (KCCQ-CSS) compared to valsartan at 8 months.55 Building on this evidence, the PARALLAX trial investigated the effect of sacubitril/valsartan versus either placebo or an ACEI/ARB (enalapril or valsartan) in patients with LVEF ≥40% and impaired quality of life (KCCQ-CSS <75 points [range 0–100]). Although treatment with sacubitril/valsartan was associated with a greater reduction in NT-proBNP levels compared to the control arm, it did not lead to significant improvements in 6 minute walk distance, KCCQ-CSS or NYHA class.57
Cyclic Guanosine Monophosphate Modulators
Besides sacubitril, which increases NP levels by inhibiting their degradation, there have been pharmacological attempts to enhance the beneficial effects of NPs by acting downstream of their cellular target, i.e. the nitric oxide (NO)-soluble guanylate cyclase (sGC)-cyclic guanosine monophosphate (cGMP) pathway.58
The NEAT-HFpEF trial assigned patients with LVEF >50% to a 6-week dose-escalation regimen of isosorbide mononitrate (ISMN; from 30 mg to 60 mg to 120 mg once daily) or placebo, with subsequent crossover to the other group for 6 weeks. Patients receiving ISMN were less active as measured by an accelerometer, with activity levels decreasing progressively with increased doses of ISMN. Moreover, there were no significant between-group differences in 6 minute walking distance, quality-of-life scores or NT-proBNP levels.59
Sildenafil is a phosphodiesterase 5 (PDE5) inhibitor, with proven efficacy in pulmonary artery hypertension. PDE5 converts second messenger cGMP into GMP and is upregulated in cardiac hypertrophy and HF.58 A small clinical trial randomised patients with HFpEF (LVEF ≥50%) and pulmonary artery systolic pressure >40 mmHg to receive sildenafil (50 mg thrice daily) or placebo. At 6 and 12 months, sildenafil significantly improved mean pulmonary artery pressure and vasomotility, right ventricular function and dimension, LV relaxation and distensibility, and lung interstitial water metabolism.60
Following these positive results, the phase III RELAX trial randomised HFpEF patients (LVEF ≥50%) with peak VO2 ≤60% of the age- and sex-specific normal value, NT-proBNP ≥400 ng/l or high LV filling pressures to either sildenafil (20 mg thrice daily uptitrated to 60 mg twice daily) or placebo. However, after 24 weeks, sildenafil did not significantly reduce peak VO2 (primary outcome), 6 minute walking distance or clinical status.61
sGC stimulators enhance sGC sensitivity to endogenous NO. sGC stimulators are a mainstay of therapy for pulmonary artery hypertension, and the sGC stimulator vericiguat has recently gained a lot of attention following the positive results of the VICTORIA trial, which led to its approval for use in patients with HFrEF.7,62
As for HFpEF, the first sGC stimulator to be investigated was riociguat, which was tested at a dose of 0.5–2 mg against placebo in the DILATE-1 trial, which included patients with LVEF >50%, mean pulmonary artery pressure ≥25 mmHg and pulmonary capillary wedge pressure >15 mmHg. After 6 hours, riociguat 2 mg failed to reduce mean pulmonary artery pressure compared to placebo (primary outcome), although it improved some exploratory haemodynamic and echocardiographic parameters, such as stroke volume, systolic blood pressure and right ventricular end-diastolic area.63
Vericiguat has been tested in two phase II RCTs on patients with HFpEF. The SOCRATES-PRESERVED trial randomised patients with LVEF ≥45% and a recent (<4 weeks) HF decompensation to receive vericiguat at various doses (1.25 mg to 10 mg daily) or placebo for 12 weeks. Treatment with vericiguat did not meet the two primary endpoints (change from baseline in NT-proBNP and left atrial volume) compared to placebo. Nonetheless, the highest vericiguat dose (10 mg daily) significantly improved the KCCQ-CCS score compared to placebo (mean difference: 9.2 points).64
This result was confirmed in a post-hoc analysis showing that both the KCCQ score and the generic health-related quality of life measure EQ-5D score had dose-dependent relationships with vericiguat.65
However, the VITALITY-HFpEF trial, which randomised patients with LVEF ≥45%, within 6 months of a recent HF decompensation and with elevated NPs to either vericiguat (10 mg or 15 mg) or placebo, failed to demonstrate an effect of vericiguat on measures of physical limitation (KCCQ-Physical Limitation Score and 6 minute walking distance) after 24 weeks of treatment.66
Neurohormonal Modulation in HFpEF: Nonpharmacological Interventions
Despite the contrasting findings of the clinical trials testing neurohormonal modulation drugs in HFpEF patients, a growing body of evidence from preclinical and clinical studies suggests that at least a subset of these patients may benefit from neuromodulation.27
The use of nonpharmacological strategies to modulate the autonomic nervous system (ANS) has therefore been proposed and tested in ancillary studies enrolling HFpEF patients, yielding encouraging results.
Renal Denervation
Considering the well-known interplay between the SNS, the RAAS, hypertension and HFpEF pathophysiology, the disruption of this link through renal denervation has been proposed as a possible therapeutic approach in HFpEF patients. Widely tested in patients with resistant hypertension, transcatheter renal denervation aims to reduce or interrupt the sympathetic innervation of renal arteries by the intravascular delivery of energy (most commonly through radiofrequency).67,68
In the RDT-PEF trial, 25 patients with HFpEF were randomised (2:1) to renal denervation versus medical therapy alone. Although renal denervation was associated with beneficial effects at 3-month follow-up, no significant differences in terms of quality of life, exercise capacity, B-type NP concentration and echocardiographic parameters were observed at 12 months.69 The underpowering of the study and the possible ineffectiveness of the ablation procedure were identified by the authors as possible explanations for the findings.69
Subsequently, Kresoja et al. compared the effects of renal denervation in patients with uncontrolled arterial hypertension with (n=99) or without (n=65) a diagnosis of HFpEF. Despite a similar efficacy in lowering blood pressure, renal denervation was associated with a greater improvement in NYHA class, NT-proBNP concentration and haemodynamic parameters (including systolic and diastolic function, aortic distensibility and myocardial work) in patients with HFpEF.70
Given these findings, renal denervation may prove valuable in the treatment of HFpEF patients. Nevertheless, optimising patient selection (e.g. focusing on those with a hypertensive phenotype) and improving ablation strategies seem necessary goals to be pursued by future clinical trials.71
Splanchnic Nerve Denervation
Inappropriate control of blood volume distribution, at rest and during physical effort, has been recently identified as a key pathophysiological determinant of HFpEF syndrome.72
Considering the key role of the autonomic modulation of visceral vessel capacitance, targeting the greater splanchnic nerve (GSN) has emerged as a potential therapeutic strategy in these patients. The surgical resection of the right GSN in 11 HFpEF patients resulted in a sustained improvement in functional capacity and quality of life by reducing cardiac filling pressure during exercise.73
More recently, the preliminary findings of the ongoing REBALANCE-HF trial have shown that, among the 18 HFpEF patients enrolled (89% in NYHA class III), the transvenous ablation of the right GSN was associated with a significant improvement in exercise filling pressures, NYHA class and quality of life in the absence of serious device/procedure-related adverse events.74 The results of the ongoing randomised, sham-controlled portion of the trial are thus expected to confirm the efficacy and the safety of this promising therapy for HFpEF patients.
Vagus Nerve Stimulation
Beyond sympathetic hyperactivity, vagal withdrawal has also been documented in HFpEF patients, potentially contributing to disease progression and poor outcomes.27 Vagus nerve stimulation (VNS) may thus provide beneficial effects in these patients.
In this regard, the ongoing ANTHEM-HFpEF study will provide information about the potential role of a device for cervical VNS in symptomatic HFpEF patients.75 However, since VNS has major drawbacks of an invasive implantation procedure and possible device-related complications, transcutaneous VNS (tVNS), carried out through the noninvasive stimulation of the auricular branch of the vagus nerve at the tragus level, is emerging as an alternative.76
In a prospective, randomised, double-blind, 2x2 crossover study, 1-hour tVNS improved heart rate variability and global longitudinal strain in the 24 HFpEF patients enrolled.77 Subsequently, in a pilot RCT including 52 HFpEF patients, 1-hour daily tVNS for 3 months improved global longitudinal strain and quality of life. Furthermore, tVNS reduced the concentration of inflammatory cytokines, underlining the important physiological link between the ANS and the immune system.78
Other Nonpharmacological Approaches in HFpEF
Beyond the strategies aimed at direct modulation of neurohormonal systems, other bioelectronic/interventional approaches have been recently tested in HFpEF.
Cardiac contractility modulation (CCM) delivers electrical impulses to the heart during the absolute refractory period, yielding beneficial structural and functional cardiac improvement, probably by increasing intracellular calcium.79 The CCM-HFpEF pilot study recently tested the effects of CCM in 47 symptomatic HFpEF patients, and CCM implantation was associated with a significant improvement in quality of life at 24 weeks, with no safety concerns arising.80 Future studies are expected to confirm these preliminary findings and evaluate the impact of CCM on hard outcomes in HFpEF patients.
An increase in left atrial filling pressure is a key pathophysiological determinant of HFpEF, contributing to poor exercise tolerance and disease progression by promoting pathological cascades.79 Lowering these pressures by creating a small shunt between the left and right atria through dedicated devices has therefore been proposed as a possible therapeutic approach. However, despite promising results in preliminary studies, the RCT REDUCE LAP-HF II has recently failed to demonstrate the clinical efficacy of this approach in a large cohort of HF patients with LVEF ≥40%, questioning the rationale behind this device.81
Neurohormonal Modulation in HFpEF: Recommendations from International Guidelines
The reasons why most trials on neurohormonal modulation therapies in HFpEF led to disappointing results are partly unclear. The intrinsic heterogeneity of HFpEF phenotype as well as flaws in study design (such as inadequate diagnostic criteria or low statistical power) might be some of the more reliable explanations.
International HF guidelines reflect this heterogeneous evidence, providing different recommendations for the management of HFpEF.7,62,82 This is due both to the different weight that the guidelines attribute to the evidence from RCTs and post-hoc analyses as well as to the different timing of their publication.
As a consequence, the 2021 European Society of Cardiology Guidelines recommend to screen for and treat underlying aetiologies and cardiovascular and non-cardiovascular comorbidities (e.g. hypertension, coronary artery disease, amyloidosis, AF and valvular heart disease) in HFpEF.7 They also recommend using diuretics to relieve HF symptoms and signs, without mentioning neurohormonal modulation therapies, stating that ‘none of the large RCTs conducted in HFpEF [had] achieved their primary endpoints’ at the time of guideline publication.7
The 2022 American College of Cardiology/American Heart Association/Heart Failure Association of America (ACC/AHA/HFSA) guidelines also underscore the importance of treating comorbidities, such as hypertension and AF, as well as the usefulness of diuretics to relieve congestion.62 However, they value more the evidence from RCTs approaching statistical significance for their primary endpoints and from post-hoc analyses showing that neurohormonal modulation therapies might be effective in specific HFpEF subgroups, especially in patients with LVEF approaching the lower boundary of HFpEF definition. Therefore, they state that ARB, MRA and sacubitril/valsartan may be considered to decrease hospitalisations in patients with LVEF ‘on the lower end of [HFpEF] spectrum’.62
This recommendation is in line with the Food and Drug Administration decision to broaden the sacubitril/valsartan indication to all patients with ‘LVEF below normal’.83 Conversely, the 2022 ACC/AHA/HFSA guidelines give an overall negative weight to the discordant results of studies on cGMP modulators, stating that ‘routine use of nitrates or phosphodiesterase-5 inhibitors to increase activity or quality of life is ineffective’ in HFpEF.62
Although a strict application of HF guidelines might create significant differences between Europe and the US, this is partly mitigated by the fact that, even without specific recommendations for HFpEF, a great proportion of HFpEF patients already receive neurohormonal modulation therapies for the treatment of underlying comorbidities.82,7 For example, in the PARAGON-HF trial, 80% of patients were receiving β-blockers at baseline – 86% received ACEI/ARBs and 26% received MRAs.55
As for non-pharmacological neuromodulation therapies, current evidence from clinical studies is still limited in patients with HFpEF, so international HF guidelines do not provide specific recommendations for their use.7,62
Neurohormonal Modulation and Sodium-glucose Cotransporter 2 Inhibitors: Future Perspectives
Numerous molecular mechanisms, including neurohormonal activation, contribute to HFpEF pathophysiology. Despite most major RCTs testing neurohormonal modulation therapies not reaching their primary endpoints, these treatments should not be definitively labelled as ineffective in HFpEF. Evidence from post-hoc analyses suggests that at least a subgroup of HFpEF patients, namely those with LVEF below normal, might derive prognostic benefit from pharmacological neurohormonal modulation, as also per the 2022 ACC/AHA/HFSA guidelines.
Although this certainly applies to ACEIs, ARBs, ARNIs and MRAs, RCT data on the use of β-blockers in HFpEF are still lacking, hence the role of this drug class in HFpEF deserves further investigation. Similarly, nonpharmacological neuromodulation therapies for HFpEF are still in their infancy and need further validation before entering the clinical arena. Conversely, cGMP modulators have been tested in several RCTs, mainly with disappointing results, casting a shadow on their efficacy in HFpEF.
Another area of uncertainty concerns the relationship between neurohormonal modulation therapies and sodium-glucose cotransporter-2 inhibitors (SGLT2Is) in HFpEF. SGLT2Is, from being antidiabetic drugs, have recently emerged as remarkable treatments with cardio- and nephroprotective effects in recent years.84
The EMPEROR-Preserved and the DELIVER trials recently demonstrated that empagliflozin and dapagliflozin reduce the combined risk of worsening HF or cardiovascular death in patients with LVEF >40%, proving to be the first drugs with a positive impact on hard cardiovascular outcomes in HFpEF.85,86 The 2022 ACC/AHA/HFSA guidelines have already endorsed the positive results of the EMPEROR-Preserved trial, recommending SGLT2Is for the treatment of HFpEF.62
Nonetheless, the mechanisms underlying SGLT2Is’ cardioprotective effects are still to be clarified. It has been proposed that SGLT2Is act as smart diuretics, promoting electrolyte-free water clearance, especially concerning the interstitial fluid space; however, this can explain only in part their positive impact on the cardiovascular system.87
In a recently published review, Packer summarised all available evidence from preclinical studies investigating the effects of SGLT2I therapy on the cardiovascular system, and concluded that, at the cellular level, SGLT2 acts as a nutrient surplus sensor, thus its inhibition through an SGLT2I causes simultaneous upregulation of nutrient-deprivation signalling and downregulation of nutrient-excess signalling, resulting in increased autophagic flux, which underlies several cytoprotective effects, such as reductions in oxidative and endoplasmic reticulum stress, restoration of mitochondrial health and improvement of mitochondrial biogenesis, and a decrease in proinflammatory and profibrotic pathways.88 A few preclinical studies have also reported an interplay between SGLT2 and the SNS, particularly at the renal level.89
Nevertheless, a direct neurohormonal modulation effect of SGLT2Is has not been demonstrated yet, hence, strictly speaking, this drug class cannot be considered a neurohormonal modulation therapy. In light of this, future studies should evaluate whether a combination of neurohormonal modulators and SGLT2Is offers any advantage over the exclusive use of the latter. While most HFpEF patients already receive β-blockers, ACEI/ARBs and MRAs to treat underlying comorbidities (for example, in the EMPEROR-Preserved trial, 86% of patients were on β-blockers at baseline, 81% were on ACEI/ARBs/ARNIs and 37% were on MRAs), sacubitril/valsartan has no indication other than HF treatment, hence the effect of the combination of SGLT2I and ARNI is still largely unexplored.85