Heart failure (HF) with preserved ejection fraction (HFpEF) is an important global health problem. HFpEF, which is mainly common in older patients with hypertension and/or obesity, is closely associated with left ventricular (LV) hypertrophy (LVH). Patients with HFpEF usually present with symptomatic HF despite a normal LV ejection fraction (LVEF) but have similar morbidity and mortality as patients with HF with reduced ejection fraction (HFrEF). An increased risk of first-onset AF and a higher incidence of stroke is also common in patients with HFpEF.1 HFpEF involves a complex interplay of pathophysiological changes involving diastolic dysfunction, remodelling of the LV and left atrium (LA), pulmonary vascular haemodynamics and non-cardiac factors, and it is associated with a poor prognosis.2–4
Diastolic properties are difficult to examine and have many determinants. It is a complex phenomenon with several phases involving both relaxation and subsequent filling of the ventricle.1 Physical examination, electrocardiography (ECG), chest radiographs, laboratory findings and multi-modality imaging methods should be used together for proper evaluation of diastolic function.1 However, no reliable and reproducible single method has been defined that can lead to a diagnosis. Invasive measurements of LV diastolic properties and pressures are impractical on a broad scale. Evaluation of the type and extent of LV diastolic dysfunction currently relies on assessment of LV filling pattern and determination of myocardial deformation with imaging tools.2–4 Although the use of multi-modality imaging is increasing – including nuclear imaging, CT and MRI – echocardiography is the first-line method for evaluation of diastolic dysfunction.2–4
Echocardiography in HFpEF
Transthoracic echocardiography (TTE) has been the standard first-line imaging modality for the evaluation of HFpEF as it is a non-invasive, widely available low-cost tool providing many strong predictors of poor prognosis.5
Transthoracic Echocardiography
A comprehensive 2D echocardiographic examination should be performed to assess diastolic properties including LV and LA dimensions, right ventricular (RV) and LV contractility, spectral Doppler properties of mitral and tricuspid valve and pulmonary vein flow, estimated pulmonary artery pressure (PAP) and tissue Doppler imaging (TDI) properties of the mitral valve annulus.5
Assessment of LV Filling
LV diastolic properties and LV filling are associated with elastic recoil, LV relaxation, LV and LA compliance, mitral valve function, viscoelasticity, LV-RV interaction, atrial contraction, the electrical system and pericardial constraint. LV filling can be basically evaluated with continuous wave (CW) and pulsed-wave (PW) Doppler techniques. LV isovolumic relaxation time (IVRT), early peak mitral flow velocity in diastole (E wave) and atrial contraction (A wave), mitral deceleration time (mitral DT) and duration of mitral A wave velocity (Adur) are commonly used Doppler parameters to assess LV filling.6
LV relaxation rate and LV compliance are substantial in assessing LV filling and pressures that indicate LV diastolic function. Doppler assessment of transmitral flow is graded as normal with impaired relaxation and pseudonormal and restrictive filling (Figure 1). Transmitral Doppler findings have a U-shaped inter-relationship with LV filling pressure. Thus, determining normal and pseudonormal patterns requires the assessment of additional echocardiographic parameters.3 Estimation of LV filling pressures is summarised in Figure 2.
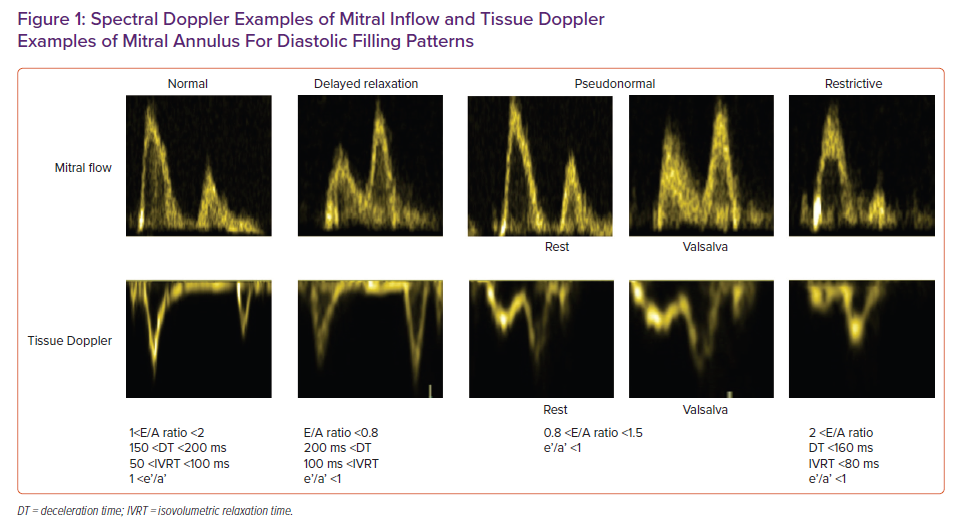
Most patients with an impaired relaxation filling pattern usually have normal filling pressures and are asymptomatic.7,8 With the progression of diastolic dysfunction, LV compliance during the atrial contraction phase decreases along with impaired LV relaxation. Decreased compliance increases mean LA pressure and dimensions. The increased LA pressure causes early mitral valve opening and higher transmitral flow velocity despite the slower LV relaxation rate, thus the LV filling pattern would be observed as normal. Patients with this pseudonormal LV filling may experience symptoms of HF and decreased exercise capacity.7–9 Significant elevation in LV pressure with severe deterioration in LV compliance causes LA dilatation and HF. Early diastolic filling becomes prominent and significant. Late diastolic filling is reduced and its duration is shortened – a restrictive filling pattern. Patients with restrictive filling usually have poor prognosis and reduced functional capacity due to HF symptoms.
Assessment of Tissue Doppler Imaging of Mitral Annulus
TDI assessment of mitral annulus is used to distinguish between normal and impaired LV filling. The ratio of the peak mitral E wave velocity to the mitral annulus velocity (E/e’) is the most used parameter.5 In addition, the mitral annulus e’/a’ ratio could also be assessed and would be >1 in most of the patients with impaired relaxation. On the other hand, the e’/a’ ratio would be <1 in patients with a pseudo-normal filling patten.10,11 The E/e’ ratio has been shown to be associated with cardiovascular endpoints. However, the relation with LV pressures has been questioned in a recent meta-analysis which reported only a moderate correlation with the invasively measured resting filling pressures.12 Still E/e’ is a guideline recommended strong echocardiographic parameter that has a prognostic value in cases of HFpEF.1
Assessment of Pulmonary Venous Flow
Pulmonary venous flow velocity assessment is performed by placing the sample of PW Doppler to the right upper pulmonary vein in apical-four chamber view. The pulmonary venous flow assessment can be used to reflect the filling haemodynamics of the LA. Doppler assessment of pulmonary venous flow is considered to be problematic in daily practice due to reproducibility and image quality, although high-quality PW Doppler transthoracic recordings can be obtained in about 85% of patients.5,13 The haemodynamic waves of PV flow rate include the peak forward flow rate in early systole (PVs1), late systole (PVs2), early diastole (PVd) and peak reverse flow rate and duration (PVa stop) in atrial contraction (PVa).14–16
Assessment of Left Atrial Function and Remodelling
Increased LA size is usually associated with high LA pressure and abnormal filling patterns. LA volume is shown to be strongly associated with adverse cardiac events and its measurement is recommended in clinical guidelines.17 Detection of normal LA dimensions usually indicates normal LA mean pressure whereas minimal volume of the LA has been observed to correlate with the pulmonary wedge pressure.5,18,19
Atrial volume and compliance are directly related to LV diastolic function which would cause LA remodelling and dysfunction. Increased LA volume occurs as a result of the chronic LV end-diastolic pressure elevation. LA volume should be calculated from the apical 4 and 2 cavity views and indexed to body surface area (LAVi). Although maximum LA volume is used more frequently, minimal LA volume can also provide important prognostic and diagnostic information.17 The upper normal limit for LAVi with 2D echocardiography is defined as 34 ml/m2. LA volumes >34 ml/m2 can be detected in 10% of the healthy population.3 The threshold for LAVi in patients with AF is >40 ml/m2 in recent guidelines.1 Enlarged LA volume is commonly observed in patients with HFpEF and is associated with increased cardiovascular risk. Therefore, LAVi should be measured in all patients with definite or suspected HFpEF.3,17
LA strain also plays an important role in the diagnosis and prognosis of HFpEF patients. Current recommendations say to evaluate LA functions by using LAVi together with LA reservoir or contractile strain for the diagnosis and prognosis of HFpEF patients.3 The novel approaches of using LA strain parameters could be fruitful in estimating LV end-diastolic pressure and implementation to conventional criteria would improve the diagnostic efficiency. LA reservoir strain should not be used for patients with poor image quality and patients with AF.5,20–23
Assessment of Tricuspid Valve Flow and Pulmonary Artery Pressure
Estimation of systolic and diastolic PAP by echocardiography is helpful when determining LV diastolic dysfunction. The end-diastolic pulmonary regurgitation rate is used to estimate diastolic PAP and the maximum velocity of the tricuspid valve regurgitation jet is used to estimate systolic PAP. It is important to evaluate and add an estimate of central venous pressure. Central venous pressure assessment is performed by right atrial pressure estimation. Inferior vena cava size and degree of respiratory collapse, hepatic venous Doppler pattern are used to assess right atrial pressure. Diastolic PAP may reflect the mean LA pressure in the absence of pulmonary vascular disease. Patients with impaired relaxation LV filling are expected to have normal or mildly increased systolic PAP whereas patients with more serious dysfunction would have increased systolic PAP associated with high LA pressure.1,2,5,24,25 Moreover, recent studies show that patients with HFpEF are at risk of developing pulmonary vascular disease which is mainly characterised by increment in pulmonary vascular resistance and reduced pulmonary arterial compliance.26 Pulmonary vascular disease can result in reduced exercise capacity and be associated with adverse outcomes.27–29 Obese patients with HFpEF are shown to have pulmonary vascular disease more often which can be sometimes manifested only during exercise.30 Mid-systolic notching in the RV outflow Doppler profile could be of value for diagnosing pulmonary vascular disease.5
Assessment of LV Dimensions and Systolic Function
Concentric or eccentric hypertrophy are common in patients with HFpEF. Both concentric remodelling and hypertrophy are associated with increased cardiovascular morbidity and mortality and commonly observed in patients with HFpEF along with eccentric hypertrophy.17 Although echocardiography is used as the first-line imaging tool, cardiac MRI (CMRI) is also useful for demonstrating fibrosis and making a differential diagnosis.3 In general, the most common cause of LV hypertrophy is hypertension, but other causes of hypertrophy should be kept in mind. When the aetiology of LV hypertrophy is unclear – and especially when echocardiographic images are insufficient to conclude the underlying pathology – CMRI is very useful for a more accurate assessment of LV structure and a more precise diagnosis. It should be remembered that LV hypertrophy in HFpEF patients cannot be an exclusion criterion because it is highly specific (88%) but weakly sensitive (26%) for the diagnosis of HFpEF.1,3,5,24,31,32
Strain Imaging in HFpEF
Deformation imaging has evolved as a promising, reproducible and valuable tool, which enables additional and better prognostic information in patients with HFpEF.33 Global longitudinal strain (GLS) is found to be reduced in more than half of the patients with HFpEF.34,35 In patients with LVH, circumferential strain and apical rotation have been shown to be increased as a compensatory mechanism to retain systolic function.17 Despite being vendor-dependent, the cut-off value would be 16%. Moreover, speckle tracking strain imaging is an excellent method for assessing diastolic function by evaluating early and late filling phasic diastolic strain rates. It reflects myocardial elongation and untwisting rate, which are closely related to diastolic function. Strain imaging can be combined with stress echocardiography and provides additional prognostic information. Pattern analysis of LV deformation can also be useful in differential diagnosis. Apical sparing is commonly observed in patients with cardiac amyloidosis, whereas longitudinal dysfunction of the septum may indicate asymmetric septal hypertrophic cardiomyopathy.34,36,37
Diastolic Stress Test by Echocardiography
Echocardiographic parameters might fail to show increased LV filling pressure signs at rest in some patients with HFpEF, however, changes in diastolic haemodynamics during exercise can lead to more sensitive assessment for diagnosing HFpEF.38 Although diastolic stress echocardiography can be performed using the supine bike or treadmill exercise protocol, supine bike is the recommended method for diastolic stress echocardiography because it allows continuous Doppler recordings throughout the exercise test.39 The regular stress test starts with 25 W workload and increases by 25 W every 3 minutes. Mitral flow, tricuspid regurgitation jet velocities and mitral annular velocities are recorded and evaluated at the start of the test, during exercise and during the recovery phase.40
A decrease in the E/A ratio or increased deceleration time of E wave is typical for mild-diastolic dysfunction or impaired myocardial relaxation. Shortening of the diastole during exercise causes an increased rate of myocardial relaxation and LV filling to provide adequate cardiac output.40 TR max velocity and E/e’ values measured during exercise were found to have high sensitivity for diastolic dysfunction and were correlated with invasive measurements.39 Stress echocardiography is also extremely useful in patients with borderline GLS. In healthy subjects, the E/e’ ratio does not change significantly with exercise due to proportional increases in mitral flow and annular velocities. In contrast, an increase in the E/e’ ratio and/or systolic PAP exercise has been shown to be correlated with increases in LV diastolic pressures.41 Septal E/e’<10 and peak tricuspid regurgitation velocity <2.8 m/s at rest and during exercise are normal findings. Septal E/e’ ratio over 15, mean E/e’ over 14 and peak TR velocity over 2.8 m/s with exercise indicates the presence of impairment in diastolic function.42 Despite being useful in evaluating increased LV filling pressures, it is difficult to record echocardiographic images with good image quality during exercise. This situation becomes more difficult with increased heart rate, but most patients with diastolic function can present diagnostic findings even at a moderately increased heart rate. If the assessment of mitral flow and annular velocities is not optimal because of immediate tachycardia, assessment of mentioned parameters during the recovery period is necessary. Stress echocardiography is one of the main methods recommended by the guidelines in the evaluation of patients with unexplained dyspnoea and subclinical LV diastolic dysfunction.21,38,41,43
CMRI in HFpEF
Given the latest criteria for the definition of HFpEF, it is obvious that to predict LV dimensions and haemodynamics with sole clinical data in HFpEF patients is impossible and performing an imaging study is inevitable.1
The aetiological work-up of patients with HFpEF would be better performed by using CMRI which is the gold standard cardiovascular imaging modality for the atrial and ventricular volume assessment and ejection fraction quantification. It is also the best alternative imaging modality in patients with suboptimal echocardiographic image quality that doesn’t only make morphological, functional evaluations but also gives information about perfusion, viability and tissue characterisation which may provide a better understanding of the pathophysiological mechanism of the HFpEF. It allows assessment of LA enlargement, LV hypertrophy, permanent replacement fibrosis and dynamic interstitial fibrosis using late gadolinium enhancement (LGE) and T1 mapping. By providing tissue characterisation with T1 and T2 mapping, CMRI is the best imaging modality in the differential diagnosis of myocarditis, infiltrative disorders, such as Fabry’s disease, sarcoidosis, both systemic and amyloid transthyretin amyloidosis, non-compaction cardiomyopathy, arrhythmogenic RV cardiomyopathy, hypertrophic cardiomyopathy, or Chagas disease.44–46 CMRI has high reproducibility and good spatial and temporal resolution. CMRI has emerged as one of the most useful techniques by minimising geometric assumptions and being less operator-dependent than other cardiovascular imaging modalities. Moreover, it is radiation-free and thus safe. New methods such as feature tracking enables myocardial strain analysis as well.
CMRI plays a pivotal role particularly in patients with obesity and lung diseases with non-diagnostic echocardiographic examinations secondary to suboptimal image quality.44 On the other hand, CMRI requires expertise in scanning and interpreting the images in the clinical context. Moreover, it is not portable and not as practical as echocardiography. Claustrophobia is one of the leading patient-related limitations of the technique. Regrettably, achieving high-resolution CMRI cine images in patients with supraventricular and ventricular arrhythmia still represents a clinical challenge. Gadolinium-based contrast agents should be used carefully in people with a glomerular filtration rate <30 ml/min/1.73 m2. However, according to recent radiology recommendations, delaying group II gadolinium-based contrast agent (GBCA) for CMRI which is clinically needed in a patient with acute kidney failure or an estimated glomerular filtration rate <30 ml/min/1.73 m2 may be more harmful than the risk of nephrogenic systemic fibrosis. Thus, the safety of using group II GBCA should be evaluated against the potential harm of delayed diagnosis.47
Assessment of LV Systolic Function
Although the LVEF is preserved, LV systolic function is not always normal in HFpEF. Impairments in LV systolic performance can be detected at rest by TTE. It has been shown that worse longitudinal strain despite preserved LVEF is a strong prognostic factor for worse outcomes in HFpEF patients. Several studies have been published showing that many patients with HFpEF have abnormal longitudinal systolic function, which can be detected by reduced mitral annulus systolic ejection velocity, mitral annulus plane systolic descent and longitudinal strain. Myocardial strain and torsion can be acquired by CMRI as well. However, most centres do not perform strain analysis routinely. This measurement is primarily a research tool which can give insights into LV systolic function. Feature tracking analysis helps to detect anatomical features of interest in the LV subendocardium and subepicardium on segmented steady-state free precession (SSFP) cine images similar to echocardiographic speckle tracking. CMRI feature tracking method provides LA strain and strain rate calculation. These measures have been demonstrated to be impaired and associated with exercise intolerance in HFpEF patients. CMRI is known to be the gold standard technique to assess biventricular morphology and systolic function, especially in patients with non-diagnostic echocardiographic studies due to bad image quality. It is the preferred imaging tool for volume and ejection fraction estimation in heart failure patients, due to its 3D approach for non-symmetrical ventricles and superior image quality which is less user-dependent and has a higher reproducibility.24,48–51 Volumes are measured from a cine stack of short-axis biventricular contiguous slices. Modern cine sequences use breath hold, electrocardiographic-gated and segmented SSFP to produce images with high-spatial/temporal resolution, which are superior to other cardiac imaging modalities. Myocardial mass is also measured from the same short axis slices and CMRI is considered the ideal method for the assessment of LV mass without geometric assumptions for the same reasons. Biventricular function is evaluated at the same time and CMRI is considered the gold-standard imaging modality for global and regional LV function as well.1,3
Assessment of LV Diastolic Function
Diastolic dysfunction is generally considered a key component for the diagnosis of HFpEF. Diastolic dysfunction diagnosis requires demonstration of elevated filling pressures. Given the invasive nature of cardiac catheterisation, it is not feasible for routine clinical use and non-invasive techniques are used for the assessment of LV diastolic function.52 LV relaxation and compliance are evaluated by measuring the transmitral inflow and pulmonary venous flow data mostly by TTE which may have several limitations such as limited field of view, cosine errors and an inadequate acoustic window.45 CMRI is another non-invasive technique which has an excellent image quality with high spatial and temporal resolution as well as great accuracy and reproducibility which may provide other various LV diastolic function parameters such as LA size and function, LV hypertrophy and mass, and myocardial deformation imaging with strain method, which are the most useful parameters for the assessment of patients with HFpEF.45,52,53
Assessment of LA
Atrial volume and function are two important measures of ventricular diastolic performance and are shown to be reliable indicators of the duration and severity of diastolic dysfunction independent from loading conditions.54 They provide significant prognostic information not only in the general population but also in patients with heart disease.55,56 LA has a poor compliance, thus LA dilation is an indicator of diastolic dysfunction with elevation of LV filling pressure in the diastolic phase. LA volume should be measured at the end of the systolic phase of the LV before the mitral valve opening. It can be measured using two methods. First, the bi-plane area-length method with manually drawn endocardial contours in 2- and 4-CH views with exclusion of pulmonary veins. Second is Simpson’s method on the short axis slices encompassing the whole left atrium. The study reported normal reference values in 108 healthy volunteers as 103 ± 30 ml for men and 89 ± 21 ml for women by area-length method.57 There is a large amount of data supporting the maximal LA volume use. However, there are also several studies demonstrating minimal LA volume to be of prognostic significance. In 140 HFpEF patientsLA emptying fraction correlated inversely with LA volumes and plasma natriuretic peptides and resulted in an independent prognostic predictor of all-cause death or hospitalisation for HF.18,58,59
Assessment of Mitral Inflow Pattern
Quantitative CMRI-derived flow measurement with phase contrast CMRI (PC-CMRI) has been used for the past four decades. It is a potentially useful alternative to echocardiographic-PW Doppler and can be used for evaluation of mitral valve flow and velocity quantification.60 An encoding velocity should be optimised to match the peak velocity as closely as possible without aliasing, which is typically 100 to 150 cm/s for the mitral inflow. After a cine-phase contrast ECG-gated CMRI sequence is performed, the slice is precisely selected using multiplanar localisation to transverse the tips of the leaflets of the mitral valve and is placed perpendicular to the LV inflow.61 This generates short-axis cine-phase contrast images. A graphical contour of the mitral valve orifice is then drawn and automatically propagated (with manual override) to all timeframes of the cine loop to calculate the velocity, peak velocity and flow plots over time.61 The E-wave peak, A-wave peak, DT and E/A ratio are calculated afterwards. Data is retrospectively ECG gated. It can be acquired using either free breathing or with a breath hold. However, the acquisition plane remains fixed during the cardiac cycle and does not accompany the cyclical motion of the mitral annulus.52 Acquisition techniques have been introduced using moving slice velocity mapping. Recently, it has been demonstrated that three-directional 3D velocity-encoded MRI with retrospective valve tracking showed better agreement with echo Doppler when differentiating a restrictive filling pattern from other patterns.52,61–63
Assessment of Pulmonary Venous Flow
Waveform analysis of pulmonary venous flow is a helpful way to assess LV diastolic dysfunction. Pulmonary vein velocity-encoded MRI is an alternative method to PW Doppler to investigate atrial filling pattern.45 2D one-directional velocity encoding at the pulmonary vein and 3D three-directional velocity-encoding approach of the intra-atrial blood flow field are two different ways of obtaining a pulmonary venous time-velocity curve. The pulmonary vein flow is sampled 1 cm into the pulmonary vein ostium similar to Doppler echocardiography. The velocity sensitivity of the acquisition should be adjusted to a maximal velocity of 80 cm/s to optimise the signal-to-noise ratio, and the acquisition plane should be perpendicular to pulmonary vein flow.45 The temporal resolution that determines the accuracy of waveform is inferior for velocity-encoded MRI when compared to echo Doppler. Moreover, it has a longer acquisition time. The use of pulmonary venous flow for the LV diastolic dysfunction assessment is limited in conditions such as sinus tachycardia, first-degree atrioventricular block and AF.60 Despite all limitations, velocity-encoded MRI offers a useful alternative to PW Doppler for pulmonary venous flow assessment and several studies have shown good correlations when compared with Doppler echocardiography.45,60
Assessment of Tissue Characterisation
Several diseases which have similar clinical presentations may have the same clinical phenotype as HFpEF and should be considered ‘phenocopies’ which confuses the diagnostic process.64 It is of utmost importance to recognise other alternative cardiac diseases, which should be considered in the differential diagnosis for HFpEF.49 Those diseases are mainly restrictive cardiomyopathy, constrictive pericarditis and severe tricuspid regurgitation. Typical CMRI findings of restrictive cardiomyopathy are biatrial dilatation and increased LV thickness.49 Sometimes small pericardial effusion can be seen as well. Diastolic function parameters are also typically impaired. Specific findings with LGE include subendocardial LV, RV free wall and septum hyperenhancement.49 Amyloidosis, cardiac sarcoidosis and haemochromatosis are the primary restrictive cardiomyopathies that are encountered in daily practice.65 CMRI is the only non-invasive technique for quantifying myocardial iron overload. Introduced in 1999, the T2* technique is a robust, fast, reproducible method that is the method of choice for cardiac iron quantification which is transferable among different CMRI scanners. T2* values in the myocardium are directly associated with iron levels in the tissue. Decreased T2* levels are associated with systolic and diastolic ventricular dysfunction; values lower than 20 ms show iron overload, while T2* lower than 10 ms indicate severe iron overload. 66–70
Cardiac amyloidosis is characterised by progressive diastolic dysfunction followed by systolic dysfunction and arrhythmia. Cardiac amyloidosis is usually diagnosed in the late stages of the disease.1,3,71
Constrictive pericarditis is another clinical scenario which can be misdiagnosed as HFpEF.49 Features of constrictive pericarditis that will be shown using CMRI include pericardial thickening, septal bounce and delayed hyperenhancement of the pericardium in patients with active inflammation.49
Tissue characterisation plays a pivotal role in the context of HFpEF in identifying specific patterns of fibrosis and scarring in most of the cardiomyopathies that could be a differential diagnosis for HFpEF (Figure 3). The identification of ‘phenocopies’ in HFPEF may allow an individualised approach to molecular targets and functional abnormalities, such as the use of some important drugs in senile amyloidosis, and ß-blockers and/or calcium channel antagonists in patients with hypertrophic cardiomyopathy.49,50,72
Novel Approaches
Although it is well known that myocardial stiffness plays an important role in cardiac function and increased stiffness may cause restrictive diastolic filling, there is still no conventional imaging method to measure myocardial stiffness directly in vivo.73,74 High-resolution magnetic resonance elastography is a novel technique based on a stiffness map produced by an external vibrating source that generates shear waves inside a tissue of interest. Arani et al. have demonstrated the feasibility of 3D high-frequency cardiac MR elastography diagnostic imaging technique for quantitatively measuring myocardial stiffness in vivo which does not require a contrast agent.74
Obesity is common in HFpEF disease and has numerous cardiovascular effects. Obokata et al. compared cardiovascular structure, function and reserve capacity in people with HFpEF and obesity and those without obesity and control subjects using echocardiography and showed that epicardial adipose tissue has a direct mechanical effect caused by increased pericardial restraint and enhanced ventricular interdependence.75 Another recent study showed the relationship between epicardial fat tissue volume and LV diastolic function, using multidetector CT and TTE, finding a significant correlation between diastolic dysfunction and increased epicardial adipose tissue.76 Epicardial adipose tissue could potentially have a pathophysiological inflammatory role in HFpEF patients, which necessitates further investigation. Considering CMRI and its ability to study anatomical structure and myocardial perfusion precisely, it may have a robust role in searching the significance of epicardial adipose tissue in the pathogenesis of HFpEF.76

Stress and exercise CMRI have emerged as important tools in HFpEF diagnosis during the past few years. Considering that patients with HFpEF develop symptoms on exertion, an exercise test for the diagnosis becomes inevitable. The gold standard for the diagnosis of the effects of HFpEF is right heart catheterisation during exercise.77 However, exercise stress echocardiography is used more frequently in daily practice because it is non-invasive.39 Additionally, stress perfusion CMRI may help identify patients at higher cardiovascular risk in HFpEF patients with no known coronary artery disease.78 Additional to that, in a study by Backhaus et al., real time-CMRI bicycle exercise stress testing showed high accuracy in the diagnosis of HFpEF, and LA longitudinal shortening during the exercise was demonstrated as the best independent predictor of HFpEF proven with an invasive method.79
4D flow is another new method which can quantitively assess LV 3D blood flow over the cardiac cycle.80 It is possible to identify and monitor diastolic dysfunction with 4D flow. High isotropic spatial resolution and low operator dependency and lack of imaging plane restriction are the principal advantages of this technique. Analysis of flow components and the kinetic energy and momentum will provide a precise assessment of the dynamic of ventricular filling and ejection.44 It can differentiate restrictive diastolic filling and normal diastolic filling patterns, and can also evaluate kinetic energy, vorticity, or particle tracing-based metrics.81,82 Machine learning and artificial intelligence (AI) are gaining importance in the medical imaging field and are expected to transform clinical practice. CMRI can use machine learning to help guide diagnosis and therapy management as it relies on complex acquisition strategies.84,85 AI is associated with radiomics which is a novel image analysis technique. Digital images are converted into numeric data which can then be analysed to obtain multiple numerical quantifiers of shape and tissue character and it has been demonstrated that disease conditions or clinical outcomes may be identified with high accuracy with this new method.86
Cardiac Computed Tomography in HFpEF
It has been already shown that cardiac CT (CCT) may provide varying parameters for the assessment of diastolic dyfunction.3,87,88 Similar to echocardiography, CCT has been applied to measure early and late diastolic filling rates for the assessment of diastolic function. However, LV filling rates are not recommended as the only indices of diastolic function due to their dependence on LV relaxation and LV filling pressure.49 Although CCT is not the first-line imaging method for comprehensive differential diagnosis of HFpEF, it may still be used as a part of multimodality imaging. One of the most useful usages of CCT is the diagnosis of constrictive pericarditis by detecting pericardial thickening. A normal EF with a concentric hypertrophy, LA enlargement and coronary artery disease (CAD) can be detected as indicators of HFpEF with CCT.49 Similar to CMRI, CCT can provide extracellular volume measurements for the further assessment of HFpEF.89
Nuclear Imaging in HFpEF
Radionuclide assessment of the systolic and diastolic function of the LV can be performed by creating an activity time plot during the cardiac cycle to obtain volumes at specific cardiac phases as the time-activity plot can be expressed as volume changes over time (end-diastolic volume (EDV/s)). This plot would provide rapid ventricular filling (peak filling rate – PFR), time to PFR, normalised PFR over to EDV/sec is normally >2.5. PFR is a sensitive marker of diastolic function; however, it can be present in young, healthy people and can show variability depending on sex, heart rate and LVEF. On the other hand, time to PFR is a more robust measure of diastolic function. Time to PFR should be normally <180 ms. Another parameter is the percentage of accumulated volume during rapid filling period. The cut-off has been shown to be 69%. Any values lower than 69% of EDV/sec during rapid filling phase would suggest diastolic dysfunction. Transmitral flow waves can also be acquired by nuclear imaging. An A/E ratio of <0.25 is suggested to be normal. Radionuclide studies are not performed for diastolic dysfunction assessment solely. However, diastolic assessment with radionuclide techniques can be added to perfusion studies. The most important radionuclide criteria suggestive of diastolic dysfunction can be listed as the peak filling rate, time to peak filling rate and transmitral flow wave ratio.90–94 Similar to CCT, nuclear methods may be helpful for the definitive diagnosis of the underlying aetiology of HFpEF. Transthyretin-type cardiac amyloidosis is common among HFpEF patients and nuclear imaging carries substantial importance for its diagnosis with high sensitivity and specifity.64,95–97 Suspected patients should be evaluated by CMRI and consequent imaging with bone scintigraphy (Figure 4). Sarcoidosis is another multisystemic disease that can involve the heart and be diagnosed with nuclear methods such as 18F-FDG-PET/CT (PET) and 123I-BMIPP/201TlCl dual myocardial single-photon emission computerized tomography.98
In summary, multimodality imaging plays a key role for defining HFpEF and establishing specific aetiology. Although echocardiography is the first-line imaging modality in patients with HFpEF, CMRI has been a cornerstone in the work-up. A simplified algorithm for the use of multimodality imaging is presented in Figure 5.
Conclusion
HFpEF is an important global health problem with increasing incidence. Diagnosis mainly depends on showing evidence of LV diastolic function and excluding other cardiac and non-cardiac pathologies. Echocardiography is the first-line imaging modality to diagnose diastolic LV dysfunction and define cardiac structure and function. Diastolic dysfunction is graded by filling pattern and filling pressure. In cases of suspected diastolic dysfunction in patients with normal filling pressures or non-conclusive echocardiographic findings, stress echocardiography should be performed. However, other clinical features should be considered as echocardiographic estimates of LV filling pressure have moderate accuracy.
CMRI is very useful for unmasking underlying pathologies in HFpEF patients. Size and function of the cardiac chambers can be easily evaluated by CMRI which provides important prognostic information. LGE and extracellular volume can add to the diagnostic work-up and prognosis by being the only imaging modality to assess myocardial fibrosis. Nuclear imaging is very useful for the diagnosis of cardiac amyloidosis. CCT and CMRI can also identify constrictive pericarditis.